Green Hydrogen Technology A Comprehensive Overview
Green hydrogen technology represents a pivotal pathway towards a sustainable energy future. Harnessing renewable energy sources like solar and wind power, electrolysis splits water into hydrogen and oxygen, creating a clean fuel with immense potential. This process, however, faces challenges in efficiency, cost, and scalability, demanding continuous innovation in electrolyzer technology and infrastructure development. Understanding these complexities is crucial to unlocking the transformative power of green hydrogen across various sectors.
From its production methods utilizing different types of electrolyzers to its storage, transportation, and diverse applications in transportation, industry, and power generation, green hydrogen offers a compelling solution to decarbonize hard-to-abate sectors. This exploration delves into the economic and policy landscapes shaping its adoption, alongside the environmental considerations and technological advancements propelling its growth.
Green Hydrogen Production Methods: Green Hydrogen Technology
Green hydrogen, produced without releasing carbon dioxide, is a crucial element in achieving a sustainable energy future. Its production relies heavily on electrolysis, a process that uses electricity to split water into its constituent elements: hydrogen and oxygen. The efficiency and cost-effectiveness of this process are key factors determining the viability of green hydrogen as a large-scale energy carrier.
Electrolysis Methods
Electrolysis is the core technology for green hydrogen production. Several types of electrolyzers exist, each with its own advantages and disadvantages. The three main categories are alkaline electrolyzers, proton exchange membrane (PEM) electrolyzers, and solid oxide electrolysis cells (SOEC). These differ primarily in the electrolyte used and the operating conditions, which significantly impact their efficiency, cost, and scalability.
Electrolyzer Type | Efficiency | Cost | Scalability |
---|---|---|---|
Alkaline Electrolyzer | 60-75% | Relatively low | High, mature technology |
PEM Electrolyzer | 70-85% | Higher than alkaline | Increasing rapidly |
SOEC Electrolyzer | 75-90% (potential) | High, currently under development | High potential, but less mature |
Renewable Energy Integration
The sustainability of green hydrogen hinges on using renewable energy sources to power the electrolysis process. Solar, wind, and hydropower are ideal candidates due to their abundance and decreasing costs. Integrating these sources directly with electrolyzers minimizes reliance on fossil fuels and reduces the overall carbon footprint.
Examples of large-scale projects integrating renewable energy and electrolysis include:
- The world’s largest green hydrogen plant in Australia, powered by wind energy, aims to produce substantial quantities of green hydrogen for export.
- Several projects in Europe are coupling solar farms with electrolyzers to produce green hydrogen for transportation and industrial applications.
- Hydropower-driven green hydrogen projects are emerging in regions with abundant hydroelectric resources, offering a reliable and sustainable source of hydrogen.
Challenges in Green Hydrogen Production
Despite significant advancements, several challenges hinder the widespread adoption of green hydrogen. Energy efficiency remains a critical factor; improving the overall efficiency of the electrolysis process is essential to reduce energy consumption and cost. The high cost of materials, particularly precious metals used in some electrolyzer types, also presents a significant barrier. Furthermore, the availability of sufficient freshwater resources for electrolysis is a concern, especially in water-stressed regions.
Addressing these challenges through technological innovation and policy support is crucial for accelerating the deployment of green hydrogen technologies.
Green Hydrogen Storage and Transportation
Green hydrogen, while offering a clean energy solution, presents significant challenges in storage and transportation due to its low energy density compared to other fuels. Efficient and cost-effective methods are crucial for its widespread adoption. This section will explore various storage and transportation options, highlighting their advantages and disadvantages, and outlining a hypothetical system for efficient delivery.
Green hydrogen technology offers a promising pathway to decarbonize various sectors, particularly heavy industry. Its production, however, heavily relies on renewable energy sources, making its success intrinsically linked to the expansion of projects like those detailed in this overview of Renewable energy projects in the US. Therefore, the growth of green hydrogen hinges on continued investment and development within the renewable energy sector.
Green Hydrogen Storage Methods
Several methods exist for storing green hydrogen, each with its own set of trade-offs. The choice of method depends on factors such as storage capacity requirements, cost, safety considerations, and the specific application.
Storage Method | Advantages | Disadvantages | Suitable Applications |
---|---|---|---|
High-Pressure Gas Storage | Relatively mature technology, lower initial capital cost compared to liquid hydrogen. | Requires robust pressure vessels, significant volume for large storage capacity, potential for leaks. | Small-scale storage, refueling stations, short-term storage near production sites. |
Liquid Hydrogen Storage | Higher energy density than gaseous hydrogen, enabling smaller storage volumes. | Requires cryogenic temperatures (-253°C), high energy consumption for liquefaction and maintenance, potential for boil-off. | Large-scale storage, long-distance transportation, industrial applications requiring significant quantities. |
Metal Hydride Storage | Safe, relatively compact storage, no need for high-pressure vessels or cryogenic temperatures. | Lower energy density compared to liquid hydrogen, relatively high cost, limited cycle life for some materials. | Portable applications, niche applications requiring safe and compact storage. |
Green Hydrogen Transportation Challenges and Opportunities
Transporting green hydrogen presents unique challenges due to its physical properties. Pipelines, cryogenic tankers, and ammonia carriers are currently being explored as viable transportation methods.Pipeline infrastructure, while established for natural gas, requires modifications to handle the unique properties of hydrogen, including its potential for embrittlement of certain materials. Cryogenic tankers offer a solution for long-distance transport, but they require significant investments in specialized infrastructure and pose challenges related to boil-off and safety.
Ammonia carriers, utilizing ammonia (NH3) as a hydrogen carrier, offer a higher energy density and easier transportation but require energy-intensive conversion processes at both ends. These methods each have opportunities for improvement and further development.
Hypothetical Green Hydrogen System: Remote Source to Industrial User
Consider a scenario where green hydrogen is produced using a remote wind farm in a sparsely populated area. The hydrogen needs to be transported to an industrial user 500km away requiring 100 tonnes of hydrogen per day.The system would comprise a 1000-tonne liquid hydrogen storage tank at the wind farm, capable of storing several days’ worth of production. This would mitigate intermittency issues associated with wind energy.
Transportation would be achieved using a fleet of specialized cryogenic tankers, each capable of carrying 50 tonnes of liquid hydrogen. Safety measures would include advanced leak detection systems, emergency shut-off valves, and rigorous driver training. The tankers would operate on a regular schedule, ensuring a consistent supply of hydrogen to the industrial user. At the industrial site, a smaller liquid hydrogen storage tank would provide a buffer before use in the industrial process.
The overall system prioritizes safety and reliability, ensuring a continuous supply of green hydrogen to meet the industrial demand.
Green hydrogen technology offers a promising pathway to decarbonize energy-intensive industries, but its widespread adoption hinges on readily available renewable energy sources for production. For homeowners seeking to contribute to this transition, exploring options like solar and wind power is crucial, as detailed in this helpful guide: Best renewable energy sources for homeowners. Ultimately, the accessibility and affordability of these renewable sources will significantly impact the future viability of green hydrogen technology.
Green Hydrogen Applications
Green hydrogen, produced without carbon emissions, offers a pathway to decarbonize numerous sectors heavily reliant on fossil fuels. Its versatility allows for integration into existing infrastructure and processes, paving the way for a cleaner energy future. This section explores key application areas, highlighting existing and planned projects demonstrating the potential of green hydrogen.
Transportation
The transportation sector presents a significant opportunity for green hydrogen adoption, particularly in heavy-duty vehicles and maritime applications where battery electric solutions face limitations. Fuel cell electric vehicles (FCEVs) offer a compelling alternative to internal combustion engines, providing comparable performance with zero tailpipe emissions.
- Fuel Cell Vehicles: Several automotive manufacturers are developing and deploying FCEVs, including Toyota’s Mirai and Hyundai’s Nexo. These vehicles utilize hydrogen fuel cells to generate electricity, offering longer ranges and faster refueling times compared to battery electric vehicles. Large-scale deployment is hampered by the current limited hydrogen refueling infrastructure.
- Heavy-Duty Transportation: Green hydrogen is ideally suited for heavy-duty applications like long-haul trucking and shipping, where battery electric solutions are less practical due to weight and energy density constraints. Companies are exploring hydrogen-powered trucks and ships, with pilot projects underway in various regions. For example, several ports are investing in hydrogen bunkering facilities to support the growth of hydrogen-powered ships.
Industry, Green hydrogen technology
Many industrial processes rely on fossil fuels, resulting in significant greenhouse gas emissions. Green hydrogen can replace these fossil fuels, offering a route to decarbonization in sectors like refining and ammonia production.
- Refining: Hydrogen is crucial in oil refining for processes like hydrocracking and hydrotreating. Replacing grey hydrogen (produced from fossil fuels) with green hydrogen can substantially reduce the carbon footprint of refineries. Several oil companies are investigating the feasibility of integrating green hydrogen into their refining operations, aiming for net-zero emissions by mid-century.
- Ammonia Production: Ammonia, primarily used in fertilizers, is currently produced using the Haber-Bosch process, a highly energy-intensive and carbon-intensive method. Green hydrogen can power a more sustainable ammonia production process, reducing reliance on fossil fuels. Projects are underway to demonstrate the viability of green ammonia production at scale, targeting the agricultural sector.
Power Generation
Green hydrogen can be used for power generation through fuel cells, offering a flexible and reliable energy source. It can also be used in power-to-gas systems, storing excess renewable energy for later use.
- Fuel Cells: Fuel cells convert chemical energy from hydrogen into electricity, offering high efficiency and zero emissions. Fuel cell power plants are being deployed in various applications, including backup power systems and distributed generation. These systems are particularly valuable in areas with intermittent renewable energy sources, providing a reliable baseload power supply.
- Power-to-Gas: Power-to-gas systems utilize excess renewable energy to produce green hydrogen through electrolysis. This hydrogen can then be stored and used later for power generation or other applications, addressing the intermittency challenges of renewable energy sources. Several large-scale power-to-gas projects are operational or under development worldwide, demonstrating the technology’s potential for grid stabilization and energy storage.
Decarbonizing Hard-to-Abate Sectors
Certain industrial sectors, such as steel production and heavy-duty transportation, pose significant decarbonization challenges due to their reliance on high-temperature processes and energy-intensive operations. Green hydrogen offers a promising pathway to reduce emissions in these sectors.
- Steel Production: The steel industry is a major emitter of greenhouse gases. Green hydrogen can replace coke in the blast furnace process, significantly reducing carbon emissions. Pilot projects are exploring the use of hydrogen-based direct reduced iron (DRI) processes, aiming to produce steel with minimal carbon footprint. The transition requires significant investment in infrastructure and technology development.
- Heavy-Duty Transportation: As mentioned earlier, green hydrogen is crucial for decarbonizing heavy-duty transportation, including long-haul trucking, shipping, and rail. The development of hydrogen refueling infrastructure and the widespread adoption of hydrogen-powered vehicles are key factors in achieving substantial emissions reductions in this sector. Government policies and incentives are crucial in driving this transition.
Economic and Policy Aspects of Green Hydrogen
The economic viability and widespread adoption of green hydrogen technology hinge on several crucial factors, including production costs, government support, and the potential for economic growth across various sectors. A comprehensive understanding of these aspects is vital for assessing the future role of green hydrogen in a sustainable energy landscape. This section will delve into the current economic realities of green hydrogen and explore the policy frameworks shaping its development.
Current Costs of Green Hydrogen Production and Future Projections
Currently, green hydrogen production is significantly more expensive than hydrogen produced from fossil fuels (grey hydrogen). The cost varies greatly depending on factors such as the specific electrolysis technology used, electricity prices, and economies of scale. Estimates place the current cost of green hydrogen production between $3 and $7 per kilogram, while grey hydrogen typically costs between $1 and $2 per kilogram.
However, substantial cost reductions are projected as technology improves and production scales up. Learning curves in manufacturing, coupled with advancements in electrolyzer efficiency and the decreasing cost of renewable energy, are expected to bring the price of green hydrogen down to levels competitive with grey hydrogen within the next decade. For instance, the International Energy Agency (IEA) projects that green hydrogen production costs could fall to below $2 per kilogram by 2030 under a scenario of ambitious policy support and technological innovation.
This cost reduction is vital for making green hydrogen a commercially viable alternative across a range of applications.
Government Policies and Incentives for Green Hydrogen Development
Governments worldwide are increasingly recognizing the strategic importance of green hydrogen and are implementing various policies and incentives to stimulate its development and deployment. These policies aim to accelerate technological advancements, reduce production costs, and create new economic opportunities.Many countries are using a multi-pronged approach, combining direct financial support with regulatory frameworks.
- The European Union: The EU’s Hydrogen Strategy aims to deploy 40 GW of renewable hydrogen electrolyzers by 2030. This is supported through substantial funding from the EU’s Innovation Fund and the NextGenerationEU recovery plan, which provide grants and loans for hydrogen projects. The EU is also developing a regulatory framework to ensure the safe and efficient integration of green hydrogen into the energy system.
- United States: The US Inflation Reduction Act includes significant tax credits for green hydrogen production, making it more economically attractive to invest in this technology. Furthermore, the Bipartisan Infrastructure Law invests billions of dollars in clean energy infrastructure, including hydrogen production and storage.
- Japan: Japan’s Green Growth Strategy prioritizes the development of a hydrogen supply chain, with government support focused on research and development, demonstration projects, and the creation of a hydrogen market. This includes funding for electrolyzer development and hydrogen infrastructure projects.
- Australia: Australia, with its abundant renewable energy resources, is positioning itself as a major exporter of green hydrogen. Government policies focus on supporting the development of large-scale renewable energy projects and hydrogen production facilities, with incentives aimed at attracting foreign investment.
Potential Economic Impact of Widespread Green Hydrogen Adoption
Widespread adoption of green hydrogen has the potential to significantly reshape various industries and the overall economy. The creation of a green hydrogen economy could lead to substantial job growth in manufacturing, construction, transportation, and energy sectors. Furthermore, the decarbonization of heavy industries, such as steel and cement production, through the use of green hydrogen could lead to significant reductions in greenhouse gas emissions, contributing to a more sustainable global economy.
For example, the transportation sector could see a substantial shift towards hydrogen-powered vehicles, reducing reliance on fossil fuels and improving air quality. Similarly, the integration of green hydrogen into the energy system could enhance energy security and resilience, particularly in regions with intermittent renewable energy sources. The overall economic impact would depend on the speed and scale of adoption, but the potential benefits are considerable, including a cleaner environment, new job creation, and enhanced energy security.
However, a successful transition requires significant investment and coordinated policy action across multiple sectors.
Environmental Impact of Green Hydrogen
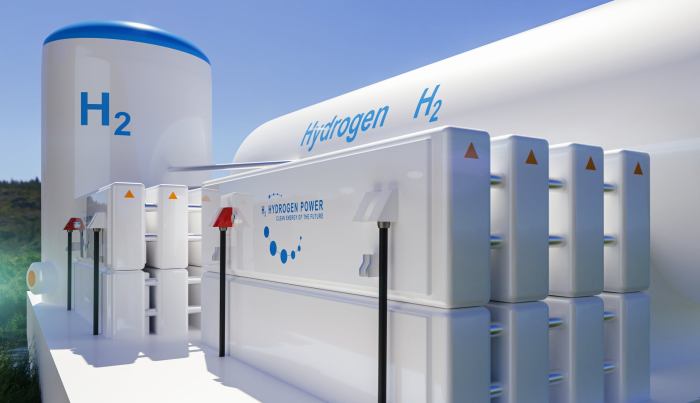
Source: renewafrica.biz
Green hydrogen, produced using renewable energy sources, offers a compelling pathway towards decarbonizing various sectors. Its environmental profile is significantly different from that of fossil fuels, presenting both substantial benefits and potential challenges that require careful consideration. A comprehensive life-cycle assessment is crucial to fully understand its overall impact.
Greenhouse Gas Emissions
The primary environmental advantage of green hydrogen lies in its exceptionally low greenhouse gas emissions during its use. Unlike fossil fuels, which release significant amounts of carbon dioxide (CO2) upon combustion, green hydrogen produces only water vapor when used in fuel cells or combustion engines. This dramatically reduces the contribution to global warming and climate change. The emissions associated with green hydrogen production, however, are highly dependent on the specific renewable energy source employed and the efficiency of the production process.
Green hydrogen technology, produced via electrolysis powered by renewable sources, offers a promising pathway to decarbonize various sectors. This clean energy solution is a key component of Green energy technologies for combating climate change , helping us transition away from fossil fuels. Further advancements in green hydrogen production and storage are crucial for its widespread adoption and impact on climate mitigation.
Air Pollution
Green hydrogen combustion generates no harmful air pollutants such as sulfur oxides (SOx), nitrogen oxides (NOx), particulate matter (PM), or carbon monoxide (CO), all of which contribute significantly to respiratory illnesses and other health problems associated with fossil fuel combustion. This clean combustion profile makes green hydrogen a particularly attractive alternative in urban areas and regions with existing air quality challenges.
Water Usage
Electrolysis, the most common method of green hydrogen production, requires substantial amounts of water. The exact water consumption varies depending on the electrolysis technology and efficiency. Concerns exist about water scarcity in certain regions, especially if large-scale green hydrogen production is concentrated in water-stressed areas. Careful site selection and the potential use of water-efficient electrolysis technologies are crucial for mitigating this impact.
Land Use Changes
The production of renewable energy needed to power electrolysis requires land for solar farms, wind farms, or hydropower facilities. This can lead to habitat loss and ecosystem disruption if not properly managed. However, the land-use intensity of renewable energy sources is generally lower than that of fossil fuel extraction, particularly coal mining. Furthermore, advancements in floating offshore wind technology and improvements in energy density can minimize the land footprint of green hydrogen production.
Resource Depletion
The production of electrolyzers and other components involved in green hydrogen production requires various raw materials, some of which are finite resources. The mining and processing of these materials can have environmental consequences, including habitat destruction, pollution, and greenhouse gas emissions. However, efforts to improve resource efficiency, use recycled materials, and explore alternative materials are crucial for minimizing these impacts.
Circular economy principles can help reduce resource depletion and waste.
Green hydrogen technology presents a significant opportunity to decarbonize various sectors. Its production, however, relies heavily on renewable energy sources, making it a key component of broader Green energy initiatives in Europe. Successful implementation requires substantial investment and coordinated policy efforts, ultimately impacting the feasibility and scalability of green hydrogen’s role in a sustainable future.
Waste Management
Green hydrogen production generates relatively little waste compared to fossil fuel extraction and processing. However, the disposal of spent electrolyzer components and other equipment requires careful management to avoid environmental pollution. Recycling and responsible disposal strategies are essential for minimizing the environmental footprint of green hydrogen technologies.
Life-Cycle Greenhouse Gas Emissions Comparison
The following table compares the life-cycle greenhouse gas emissions of green hydrogen with other low-carbon energy sources. Note that these values can vary significantly depending on the specific production methods, location, and assumptions used in the life-cycle assessment.
Energy Source | Greenhouse Gas Emissions (gCO2e/kWh) | Production Method | Notes |
---|---|---|---|
Green Hydrogen (Electrolysis) | 4-12 | Electrolysis powered by renewable energy | Highly variable depending on renewable energy source and electrolysis efficiency. |
Nuclear Power | 12-15 | Nuclear fission | Low greenhouse gas emissions, but concerns about nuclear waste disposal. |
Solar Photovoltaic | 40-50 | Solar panels | High upfront energy requirements for manufacturing. |
Onshore Wind | 10-12 | Wind turbines | Relatively low emissions, but land use considerations. |
Technological Advancements in Green Hydrogen
The pursuit of clean energy necessitates continuous innovation in green hydrogen production. Recent advancements across various technological fronts are significantly improving the efficiency, reducing the costs, and enhancing the durability of green hydrogen production methods, paving the way for wider adoption. This section will explore key advancements in electrolyzer technology, the role of materials science and nanotechnology, and the application of artificial intelligence and machine learning in optimizing the entire green hydrogen value chain.Electrolyzer technology is undergoing a rapid transformation.
Improvements in efficiency are being driven by the development of new catalysts and membrane materials, allowing for higher current densities and lower energy consumption. Cost reductions are being achieved through economies of scale, streamlined manufacturing processes, and the utilization of less expensive materials. Durability enhancements focus on increasing the lifespan of electrolyzers, reducing maintenance requirements, and improving their resilience to operational challenges.
Electrolyzer Technology Advancements
Significant progress is being made in various electrolyzer types. Alkaline electrolyzers, while mature technology, are benefiting from improved designs and materials, leading to increased efficiency and lower costs. Proton exchange membrane (PEM) electrolyzers are seeing advancements in membrane and catalyst technologies, resulting in higher operating pressures and improved efficiency, particularly at smaller scales. Solid oxide electrolyzer cells (SOECs), operating at high temperatures, offer the potential for even higher efficiencies but face challenges related to materials durability and cost.
For example, researchers at the National Renewable Energy Laboratory (NREL) have demonstrated a PEM electrolyzer with a record-high efficiency exceeding 80%, showcasing the potential for significant advancements in this field. This improvement is largely attributed to the development of novel catalyst materials with enhanced activity and durability. Furthermore, advancements in bipolar plate designs are minimizing energy losses and improving overall system efficiency.
Materials Science and Nanotechnology’s Role
Materials science and nanotechnology are crucial in driving down the cost and enhancing the performance of green hydrogen production. The development of novel catalyst materials with increased activity and durability is paramount. Nanomaterials, such as nanoparticles and nanowires, offer a large surface area for catalytic reactions, enhancing the efficiency of the electrolysis process. For instance, the use of platinum-group metal-free (PGM-free) catalysts is a significant area of research, aiming to replace expensive platinum-based catalysts with more affordable alternatives, like nickel-iron alloys.
Improved membrane materials with enhanced proton conductivity and chemical stability are also critical for improving electrolyzer performance and lifespan. Research into novel polymer materials and ceramic membranes is leading to significant breakthroughs in this area.
Artificial Intelligence and Machine Learning Applications
Artificial intelligence (AI) and machine learning (ML) are increasingly being used to optimize green hydrogen production and distribution. ML algorithms can analyze vast amounts of data from electrolyzer operation to predict maintenance needs, optimize operating parameters for maximum efficiency, and detect anomalies that could lead to equipment failure. AI-powered predictive models can forecast energy demand and optimize hydrogen production to match fluctuating renewable energy sources, maximizing the utilization of intermittent renewables.
Furthermore, AI can be used to optimize the design of electrolyzers, leading to improved performance and reduced costs. Real-world applications are already emerging, with companies using AI to control and monitor their electrolyzer fleets, improving operational efficiency and reducing downtime. For example, some companies are using ML models to predict the remaining useful life of electrolyzer components, allowing for proactive maintenance and minimizing unexpected shutdowns.
Final Summary
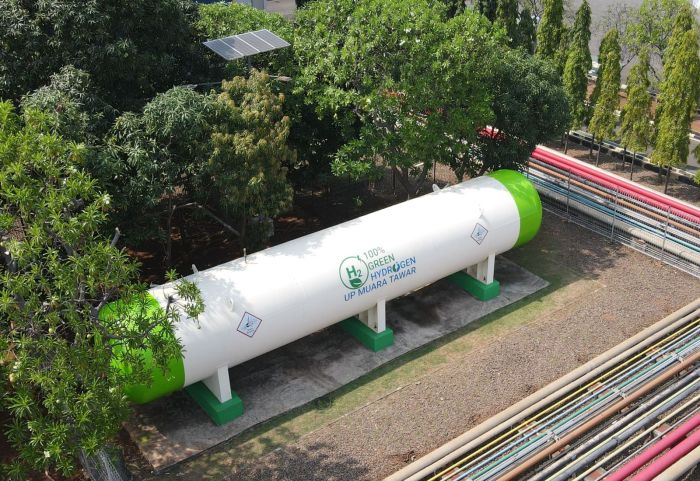
Source: fokuspapua.com
Green hydrogen technology, while facing hurdles, presents a promising pathway to a cleaner energy future. The ongoing advancements in electrolyzer efficiency, coupled with supportive government policies and the growing recognition of its potential across numerous sectors, paint a picture of significant future growth. Overcoming challenges in cost, scalability, and infrastructure will be critical to realizing the full potential of green hydrogen as a key player in global decarbonization efforts.
The transition requires collaborative efforts across research, industry, and policy to fully unlock this clean energy source’s transformative capabilities.
Essential FAQs
What are the main environmental concerns surrounding green hydrogen production?
While green hydrogen itself is emission-free when used, its production can have environmental impacts. These include land use for renewable energy infrastructure, water consumption in electrolysis, and potential resource depletion related to electrolyzer materials. Careful site selection and sustainable resource management are vital to mitigate these concerns.
How does green hydrogen compare to other renewable energy sources like solar and wind?
Unlike solar and wind power, which are intermittent, green hydrogen can be stored and transported, offering energy storage and flexibility. This makes it ideal for balancing renewable energy supply and demand and powering sectors difficult to electrify directly.
What role does government policy play in the development of green hydrogen?
Government policies, including subsidies, tax incentives, and regulations, are crucial for driving down the cost of green hydrogen production, stimulating investment in infrastructure, and creating a market for green hydrogen applications. These policies vary across countries, but generally aim to accelerate the transition to a low-carbon economy.
What are the safety considerations associated with hydrogen storage and transport?
Hydrogen is flammable, so safety protocols for storage and transport are paramount. These include robust containment systems, leak detection mechanisms, and specialized training for personnel handling hydrogen. The specific safety measures depend on the storage and transportation method employed (e.g., high-pressure tanks, cryogenic tankers).