Floating Solar Farms A Sustainable Energy Solution
Floating solar farms, also known as floatovoltaics, represent a burgeoning field in renewable energy, offering a unique approach to harnessing solar power. These systems utilize large arrays of solar panels mounted on floating platforms, typically situated on bodies of water such as reservoirs, lakes, and coastal areas. This innovative approach addresses land scarcity concerns while potentially offering environmental benefits, making it a compelling solution for expanding renewable energy infrastructure.
The technology behind floating solar farms is constantly evolving, incorporating advancements in panel design, mooring systems, and grid integration. Economic considerations, including cost-effectiveness and job creation, also play a significant role in their feasibility and adoption. This exploration delves into the various aspects of floating solar farms, examining their environmental impact, technological advancements, economic viability, and future prospects.
Environmental Impact of Floating Solar Farms
Floating solar farms, while offering a promising renewable energy solution, present a complex interplay of environmental benefits and potential drawbacks. Their impact on aquatic ecosystems and water quality requires careful consideration to ensure sustainable deployment and minimize negative consequences. This section details the environmental considerations associated with these innovative power generation systems.
Water Quality Impacts
The introduction of floating solar farms into water bodies can influence water quality in several ways. One concern is the potential for increased algae growth. The shading provided by the solar panels can alter light penetration, potentially leading to changes in the phytoplankton community. While some studies suggest minimal impact, others highlight the possibility of localized changes in algae species composition and abundance, depending on factors such as water depth, nutrient levels, and the density of the solar farm.
Furthermore, changes in water temperature can occur beneath the panels due to reduced sunlight penetration and altered water circulation patterns. These temperature changes could affect the thermal stratification of the water column and impact aquatic organisms sensitive to temperature fluctuations. The extent of these effects is dependent on the size and design of the floating solar farm, as well as the specific characteristics of the water body.
For example, a large farm in a shallow, eutrophic lake might experience more pronounced changes than a smaller farm in a deep, oligotrophic reservoir.
Impacts on Aquatic Ecosystems
Floating solar farms can directly and indirectly affect aquatic ecosystems. The physical presence of the panels alters the surface habitat, potentially disrupting the natural environment for some species. Birds and other wildlife might find their foraging or nesting areas altered. Furthermore, changes in water quality, such as altered light penetration and temperature, can cascade through the food web, impacting various aquatic organisms, from phytoplankton to fish and other larger animals.
However, it is important to note that some studies have suggested that floating solar farms can also create new habitats, providing shade and shelter for certain aquatic species. The overall impact on biodiversity is likely to be context-specific, depending on the design and location of the farm, and the pre-existing ecosystem characteristics. For instance, a well-designed floating solar farm integrated with ecological considerations could provide beneficial habitat for some species, while a poorly designed or inappropriately sited farm could cause significant disruption.
Carbon Footprint Comparison
Comparing the carbon footprint of floating solar farms to land-based solar farms requires a life-cycle assessment (LCA) considering manufacturing, installation, operation, and decommissioning. While both technologies share similar manufacturing processes, the installation of floating farms may involve additional material use for the floating platforms and anchoring systems. However, floating solar farms may sometimes have a lower land-use impact than land-based farms, potentially offsetting some of the additional material requirements.
Operational impacts, such as energy loss and maintenance, are relatively similar between the two types. Several studies have shown that the overall carbon footprint of floating solar farms can be comparable to or even lower than that of land-based systems, particularly in regions with limited suitable land for solar development. For example, a study conducted in [insert location of study] showed that a floating solar farm had a [insert percentage] lower carbon footprint compared to a land-based solar farm of equivalent capacity, primarily due to reduced land-use change.
Environmental Impact Comparison Table
Impact Category | Floating Solar | Wind Energy | Hydroelectric |
---|---|---|---|
Water Quality | Potential for algae growth changes, temperature changes | Minimal direct impact, potential for erosion near turbines | Significant alteration of flow regime, water temperature, sedimentation |
Ecosystem Impact | Habitat alteration, potential for both positive and negative effects on biodiversity | Bird mortality, habitat fragmentation, noise pollution | Habitat loss, changes in fish migration patterns, altered water quality |
Land Use | Low, utilizes water surface | Moderate, requires land for turbine placement and infrastructure | High, requires dam construction and reservoir creation |
Carbon Footprint | Comparable to or potentially lower than land-based solar | Relatively low, but depends on manufacturing and material sourcing | Relatively low, but varies greatly depending on dam size and location |
Technological Aspects of Floating Solar Farms
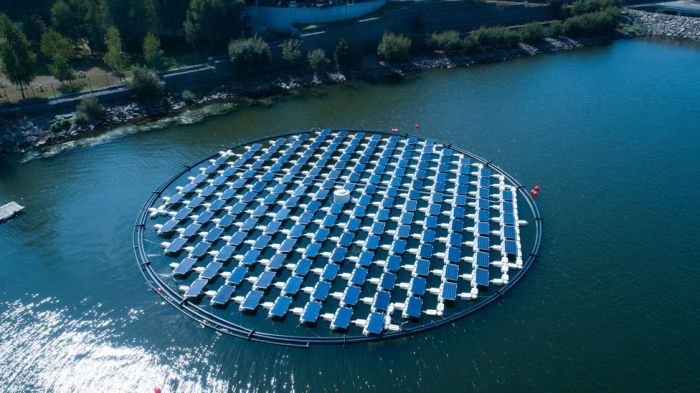
Source: co.uk
Floating solar farms, also known as floatovoltaics, represent a significant advancement in renewable energy technology. Their unique design presents a range of technological challenges and innovations, from the construction of the floating platforms themselves to the intricate electrical systems required for grid connection. This section details the key technological components and advancements in this rapidly evolving field.
Floating Platform Types
Several types of floating platforms are employed for solar panel installation, each with its own strengths and weaknesses depending on factors such as water depth, wave action, and cost. The choice of platform significantly impacts the overall stability and longevity of the system. Common types include:
- Pontoon-based platforms: These utilize interconnected buoyant modules, often made from high-density polyethylene (HDPE) or other durable, buoyant materials. They are relatively simple to construct and deploy, making them cost-effective for many applications. Their modular design allows for scalability and easy maintenance.
- Modular floating structures: These are prefabricated units that are assembled on-site. They can be designed to withstand harsher environmental conditions and are often more robust than pontoon systems. This approach allows for customization and efficient deployment in challenging environments.
- Specialized floating structures: This category encompasses platforms designed for specific water conditions or project requirements. Examples include platforms designed for deep water or those incorporating advanced wave dampening technologies. These often represent a higher initial investment but may offer long-term advantages in specific circumstances.
Mooring Systems
The mooring system is crucial for maintaining the stability and positioning of the floating solar farm. Its effectiveness directly influences the operational life and performance of the entire system. Several mooring techniques are used, each tailored to specific water conditions and environmental factors.
- Anchored systems: These involve securing the floating platform to the seabed using anchors and chains or ropes. This method is effective in relatively calm waters but can be challenging in deeper waters or areas with strong currents.
- Dynamic positioning systems: These utilize GPS and thrusters to maintain the platform’s position, offering greater flexibility and adaptability in dynamic water conditions. However, they require more sophisticated technology and higher operational costs.
- Combination systems: Many floating solar farms employ a combination of anchoring and dynamic positioning to optimize stability and cost-effectiveness. This approach allows for adaptation to varying water conditions and reduces reliance on a single mooring method.
Electrical Systems and Grid Connection
The electrical system is responsible for collecting the power generated by the solar panels and transmitting it to the grid. This involves a complex interplay of underwater cables, substations, and grid integration strategies. High voltage cables are commonly used to minimize transmission losses over long distances. Substations on land or floating substations are employed to step up the voltage before transmission to the main grid.
Sophisticated monitoring and control systems are integrated to ensure efficient energy distribution and grid stability. The design and implementation of these systems require careful consideration of safety, reliability, and environmental factors.
Innovative Technologies for Efficiency and Durability
Continuous research and development are driving improvements in the efficiency and durability of floating solar farms. Several innovative technologies are being implemented to address challenges related to water exposure, corrosion, and performance optimization. These include:
- Self-cleaning solar panels: These panels incorporate coatings or designs that minimize the accumulation of dirt, algae, and other debris, maximizing energy production over time.
- Advanced materials: The use of corrosion-resistant materials and improved panel encapsulation techniques enhances the longevity and durability of the floating solar farm, reducing maintenance costs and extending the operational lifespan.
- Smart monitoring and control systems: These systems leverage data analytics and artificial intelligence to optimize energy production, predict maintenance needs, and enhance the overall efficiency of the floating solar farm. Real-time monitoring allows for immediate response to potential issues.
Economic and Social Considerations
Floating solar farms present a compelling blend of environmental sustainability and economic viability, offering a unique pathway towards a cleaner energy future. Their implementation, however, is not without its complexities, involving a multifaceted interplay of economic incentives, regulatory hurdles, and public perception. A thorough understanding of these factors is crucial for successful project development and widespread adoption.
Economic Benefits of Floating Solar Farms
Floating solar farms offer several significant economic advantages. The most immediate benefit is the potential for substantial cost reductions in electricity generation. By utilizing otherwise unusable water bodies, they eliminate the need for expensive land acquisition, a major cost driver in traditional solar projects. Furthermore, the cooling effect of the water improves the efficiency of solar panels, leading to increased energy output.
The construction and operation of these farms also create numerous jobs, encompassing roles in engineering, construction, maintenance, and operations. For example, the development of a large-scale floating solar farm could create hundreds of jobs in a local community, stimulating economic growth and providing employment opportunities. These projects also often involve partnerships with local businesses, further enhancing the economic impact.
The reduced reliance on fossil fuels translates to long-term savings for consumers and businesses alike, bolstering energy independence and security.
Challenges in Land Acquisition and Permitting
Despite the economic allure, navigating the regulatory landscape can pose significant challenges. Securing the necessary permits and approvals for floating solar farm projects often involves a complex and time-consuming process, requiring interaction with various government agencies and stakeholders. This includes obtaining permits for water usage, environmental impact assessments, and navigating local zoning regulations. Negotiating lease agreements with landowners or water management authorities can also be protracted, potentially delaying project timelines and increasing overall costs.
The lack of standardized permitting processes across different regions further complicates the matter, making it crucial to have experienced legal and regulatory experts involved from the project’s inception. For instance, a project delayed due to permitting issues can lead to increased financial burdens and potentially jeopardize the project’s feasibility.
Floating solar farms represent a promising avenue for expanding renewable energy capacity, particularly in water-rich regions. These innovative projects are attracting significant investment, and are a key component of Green energy investments in Australia , which are driving substantial growth in the sector. The potential for large-scale deployment of floating solar farms makes them a vital part of Australia’s renewable energy future.
Social Acceptance and Public Perception
Public perception and social acceptance play a crucial role in the successful implementation of floating solar farms. In some communities, there may be concerns about the visual impact of the farms on the landscape, potential effects on aquatic ecosystems, or impacts on recreational activities. Open and transparent communication with local communities, addressing these concerns proactively through public forums and educational initiatives, is vital to fostering acceptance.
Successful projects often involve community engagement strategies that emphasize the environmental benefits, economic opportunities, and minimal disruption to local ecosystems. A positive public perception can significantly ease the permitting process and ensure the long-term success of these projects. Conversely, negative public opinion can lead to delays, increased costs, and even project abandonment. For example, a project facing significant public opposition might require extensive mitigation measures, adding to the overall expense.
Economic Advantages and Disadvantages Compared to Traditional Energy Sources
The following points summarize the economic advantages and disadvantages of floating solar farms when compared to traditional energy sources like fossil fuels and land-based solar farms:
- Advantages: Lower land acquisition costs, increased energy efficiency due to water cooling, job creation in construction and operation, reduced electricity costs for consumers, potential for revenue generation through power purchase agreements.
- Disadvantages: Higher initial capital investment compared to some fossil fuel plants, potential for longer permitting and approval processes, dependence on water availability and quality, challenges in maintenance and repair in harsh weather conditions, potential for environmental impacts if not properly managed.
Feasibility and Suitability of Locations
The successful deployment of a floating solar farm hinges critically on the careful selection of a suitable location. Several geographical and environmental factors must be considered to ensure both the operational efficiency and the long-term viability of the project. A thorough site assessment is paramount to mitigate potential risks and maximize energy generation.Geographical factors significantly influence the suitability of a location for a floating solar farm.
Floating solar farms offer a compelling solution for maximizing renewable energy generation, especially in water-rich regions. This innovative approach complements other renewable energy sources, such as the substantial growth seen in Wind energy farms in Asia , which are similarly focused on sustainable power. Ultimately, the diversification of renewable energy portfolios, including both floating solar and wind power, is crucial for a secure and sustainable energy future.
These factors interact in complex ways, requiring a holistic assessment approach.
Geographical Factors Influencing Site Suitability
Water depth, water currents, sunlight availability, and wave action are key considerations. Shallow water is generally preferred to reduce the complexity and cost of anchoring systems. Strong currents can increase the stress on the floating structures and mooring systems, necessitating robust designs. Areas with high sunlight irradiance are essential for maximizing energy production. High wave action can impact the stability of the floating platforms and potentially damage the solar panels.
Furthermore, the presence of aquatic life and the potential impact on the ecosystem needs careful consideration.
Site Assessment and Selection Process
The site assessment process typically involves several stages. Initially, a preliminary screening identifies potential locations based on readily available data, such as bathymetry maps, solar irradiance data, and wind speed information. This is followed by detailed site surveys to gather specific data on water depth, currents, wave height, and water quality. Environmental impact assessments are crucial at this stage to identify and mitigate potential ecological consequences.
Finally, a detailed engineering assessment determines the feasibility of constructing and operating a floating solar farm at the selected location, considering factors such as anchoring systems, cable routing, and grid connection. The assessment will also consider the costs associated with each potential location. For example, a site requiring extensive dredging or specialized anchoring systems will be more expensive than a site with naturally favorable conditions.
Feasibility in Different Water Bodies, Floating solar farms
Floating solar farms can be deployed in various water bodies, each presenting unique challenges and opportunities. Reservoirs often provide a stable environment with relatively calm waters and existing infrastructure, making them attractive locations. Lakes, depending on their size and depth, can also be suitable, but the absence of existing infrastructure might increase the project’s cost. Coastal waters, while offering vast areas, present significant challenges due to wave action, salinity, and potential marine interactions.
The feasibility is strongly influenced by the specific characteristics of each water body. For example, a large, deep lake with significant wave action might be less suitable than a smaller, shallower reservoir with calmer waters.
Factors Influencing Feasibility of Floating Solar Farms
Factor | Description | Impact | Mitigation Strategy |
---|---|---|---|
Water Depth | Depth of the water body | Influences anchoring system design and cost | Select shallow water locations or employ specialized anchoring systems |
Water Currents | Strength and direction of water currents | Affects structural stability and mooring system design | Use robust mooring systems and design floating platforms to withstand currents |
Sunlight Availability | Amount of solar irradiance received | Determines energy generation potential | Select locations with high solar irradiance and optimize panel orientation |
Wave Action | Height and frequency of waves | Impacts structural integrity and operational stability | Design floating platforms with sufficient buoyancy and employ wave attenuators |
Water Quality | Presence of pollutants or algae | Can affect panel efficiency and lifespan | Regular cleaning and maintenance of panels |
Environmental Impact | Effects on aquatic life and ecosystems | Potential negative impacts on biodiversity | Conduct thorough environmental impact assessments and implement mitigation measures |
Grid Connection | Proximity to the electricity grid | Affects transmission costs and efficiency | Optimize cable routing and consider energy storage solutions |
Regulatory Framework | Permits and approvals required | Can delay project implementation and increase costs | Engage with regulatory authorities early in the project planning |
Maintenance and Operation: Floating Solar Farms
The successful operation of a floating solar farm hinges on a robust and proactive maintenance strategy. Unlike terrestrial solar farms, the aquatic environment introduces unique challenges requiring specialized techniques and technologies for efficient upkeep and optimal energy production. Regular maintenance minimizes downtime, extends the lifespan of the system, and ensures consistent energy output.
Maintenance procedures for floating solar farms encompass a range of activities, from routine cleaning and inspections to more complex repairs and system upgrades. The frequency and intensity of these activities depend on several factors, including environmental conditions (weather, water quality), the age of the system, and the specific design of the floating platform and solar panels.
Cleaning Procedures
Regular cleaning of solar panels is crucial for maximizing energy generation. Accumulation of dirt, debris, bird droppings, and algae can significantly reduce the efficiency of the panels. Cleaning methods may involve manual wiping, high-pressure water jets, or specialized robotic cleaning systems. The choice of method depends on the scale of the farm, accessibility, and the type of soiling.
For instance, large-scale farms might utilize automated cleaning systems incorporating drones or specialized boats equipped with cleaning apparatus, while smaller farms may rely on manual cleaning. The frequency of cleaning depends on local environmental conditions; coastal areas with higher levels of salt spray might require more frequent cleaning than inland locations.
Repair and Inspection Procedures
Regular inspections are essential to identify and address potential issues before they escalate into major problems. These inspections involve visual checks for damage to the panels, floating platforms, mooring systems, and electrical components. Infrared thermography can be used to detect hot spots indicative of faulty connections or damaged cells. Drones equipped with high-resolution cameras provide a cost-effective way to inspect large areas quickly.
Floating solar farms offer a unique solution to land scarcity, maximizing renewable energy generation. Their electricity output can be directly utilized to power the electrolysis process integral to Green hydrogen technology , creating a sustainable energy ecosystem. This synergy allows floating solar farms to contribute significantly to a cleaner energy future, minimizing reliance on fossil fuels.
Repairs may involve replacing damaged panels, repairing or replacing mooring lines, and addressing electrical faults. A comprehensive maintenance schedule should incorporate both preventative and corrective maintenance strategies to ensure the long-term performance of the floating solar farm.
Challenges in Accessing and Maintaining Floating Solar Panels
Accessing and maintaining floating solar panels presents unique challenges compared to terrestrial installations. The aquatic environment necessitates specialized equipment and procedures. Boat access is often required, weather conditions can significantly impact accessibility, and the potential for water damage to equipment needs careful consideration. Safety protocols must be stringent, incorporating appropriate personal protective equipment (PPE) and safety training for personnel involved in maintenance tasks.
The design of the floating platform should also facilitate easy access for maintenance and repair. For example, walkways or designated access points can improve the efficiency and safety of maintenance operations.
Remote Monitoring and Control Technologies
Remote monitoring and control systems play a vital role in optimizing the operation and maintenance of floating solar farms. These systems use sensors and data acquisition units to collect real-time data on various parameters, including solar panel performance, water level, weather conditions, and system health. This data is transmitted to a central control system, allowing operators to monitor the farm’s performance remotely and identify potential problems early.
Examples of technologies used include SCADA (Supervisory Control and Data Acquisition) systems, IoT (Internet of Things) sensors, and advanced analytics platforms. Predictive maintenance models, utilizing machine learning algorithms, can be developed to forecast potential failures and optimize maintenance schedules. This approach reduces downtime and minimizes maintenance costs.
Maintenance and Operation Flowchart
The flowchart would depict a sequential process, starting with regular inspections (visual checks, infrared thermography, drone surveys), leading to the identification of maintenance needs. This branches into corrective maintenance (repairing damaged panels, replacing components, addressing electrical faults) and preventative maintenance (cleaning, system upgrades). The process would also include data logging and analysis from remote monitoring systems, feeding back into the inspection phase to inform future maintenance schedules.
A feedback loop would ensure continuous improvement and optimization of the maintenance strategy. The flowchart would visually represent the iterative nature of the maintenance and operation cycle, highlighting the integration of remote monitoring and control technologies.
Future Trends and Developments
Floating solar farms, while already a burgeoning technology, are poised for significant advancements in efficiency and scalability. The next generation of these systems will likely incorporate innovative materials, smarter designs, and deeper integration with other renewable energy sources, paving the way for a more sustainable and resilient energy future.Emerging technologies promise to significantly enhance the efficiency and scalability of floating solar farms.
These improvements will focus on maximizing energy production while minimizing environmental impact and operational costs.
Floating solar farms, also known as floatovoltaics, represent a promising innovation in renewable energy generation. These systems offer a compelling solution by harnessing solar power while conserving valuable land resources, unlike traditional ground-mounted solar farms. This aligns perfectly with the broader goals of Green energy technologies for combating climate change , and further contributes to mitigating the impacts of climate change.
The potential for large-scale deployment of floating solar farms is significant, offering a sustainable and efficient pathway towards a cleaner energy future.
Enhanced Solar Panel Technology
The efficiency of floating solar farms is directly tied to the performance of the solar panels themselves. Future developments will likely see widespread adoption of perovskite solar cells, which offer higher efficiency rates than traditional silicon-based panels, even under low-light conditions. Furthermore, advancements in bifacial solar panels – capable of absorbing light from both sides – will further boost energy generation by utilizing reflected sunlight from the water’s surface.
Research into flexible and lightweight solar panels could also lead to easier installation and reduced transportation costs, making floating solar farms more economically viable in remote locations. For instance, the ongoing research into perovskite-silicon tandem cells shows promising results in significantly improving the overall energy conversion efficiency, potentially exceeding 30%.
Integration with Other Renewable Energy Technologies
The synergistic potential of combining floating solar farms with other renewable energy technologies is substantial. A prime example is the integration with wave energy converters. Wave energy systems, situated beneath or alongside the floating solar farm, can provide a consistent baseload power supply, supplementing the intermittent nature of solar energy. Similarly, integrating floating wind turbines within or near the solar farm creates a hybrid renewable energy system, further diversifying energy production and enhancing grid stability.
A real-world example could be a coastal location where the existing wave energy infrastructure is upgraded to accommodate floating solar panels, creating a multi-faceted renewable energy hub.
Hypothetical Future Floating Solar Farm: “Oceanus Solis”
Imagine “Oceanus Solis,” a large-scale floating solar farm located in a sheltered coastal bay. This hypothetical farm utilizes advanced perovskite-silicon tandem solar panels mounted on a modular, self-adjusting floating platform. These platforms are equipped with sensors that monitor water levels, wave activity, and solar irradiance, allowing for real-time adjustments to optimize energy capture. Beneath the solar panels, wave energy converters capture the kinetic energy of ocean waves, providing a consistent energy supply even during periods of low sunlight.
The entire system is connected to an onshore grid via underwater cables, with integrated energy storage solutions – such as advanced flow batteries – managing energy fluctuations and ensuring a stable power supply. Excess energy is used to power desalination plants, providing freshwater to nearby communities.
Visual Representation of Oceanus Solis
Oceanus Solis is envisioned as a vast expanse of interconnected, hexagonal floating platforms, each supporting a dense array of high-efficiency perovskite-silicon tandem solar panels. The platforms are anchored to the seabed using a combination of mooring lines and ballast systems, ensuring stability even in moderately rough seas. Beneath the solar panels, cylindrical wave energy converters are subtly integrated into the platform design, almost invisible from above.
The overall appearance is one of sleek, modern technology seamlessly integrated into the marine environment, with the solar panels reflecting the sunlight and creating a shimmering, almost ethereal effect on the water’s surface. The farm is surrounded by smaller, autonomous maintenance drones that regularly inspect and clean the panels, ensuring optimal performance. At the edge of the farm, wind turbines rise elegantly from the water, completing the hybrid renewable energy system.
Conclusion
In conclusion, floating solar farms present a promising pathway towards a more sustainable energy future. While challenges related to environmental impact, technological hurdles, and economic factors remain, ongoing research and development are steadily addressing these concerns. The potential for integrating floatovoltaics with other renewable energy sources, coupled with their adaptability to various geographical locations, positions them as a significant contributor to the global transition towards cleaner energy.
Further exploration and investment in this technology are crucial for realizing its full potential.
Key Questions Answered
What are the maintenance requirements for floating solar farms?
Maintenance involves regular cleaning of panels, periodic inspections for damage, and repairs as needed. Specialized equipment and techniques are often required due to the aquatic environment.
How do floating solar farms impact water quality?
Potential impacts include changes in water temperature and shading effects that can influence aquatic life. Careful site selection and monitoring are crucial to minimize negative consequences.
What are the insurance implications of floating solar farms?
Insurance coverage needs to account for unique risks associated with the aquatic environment, including weather events and potential damage from waterborne debris. Specialized insurance policies are typically required.
Are there any regulations specific to floating solar farms?
Regulations vary by location and may address environmental permits, safety standards, and grid connection requirements. It’s crucial to comply with all relevant local, regional, and national regulations.