Renewable Energy Storage Solutions A Comprehensive Overview
Renewable energy storage solutions are crucial for harnessing the intermittent nature of solar and wind power. Their efficient implementation is vital for a sustainable energy future, requiring advancements in technology, supportive policies, and careful integration into existing power grids. This exploration delves into the various types of storage, their technological advancements, economic considerations, environmental impacts, and future prospects, providing a comprehensive understanding of this rapidly evolving field.
From pumped hydro to cutting-edge battery technologies, the landscape of renewable energy storage is dynamic and complex. Understanding the advantages and disadvantages of each approach, along with the challenges of grid integration and the environmental considerations, is paramount to building a truly sustainable energy system. This overview aims to illuminate the key aspects of this critical technology, paving the way for informed discussions and strategic decision-making.
Types of Renewable Energy Storage: Renewable Energy Storage Solutions
Renewable energy sources, such as solar and wind, are inherently intermittent. Their output fluctuates depending on weather conditions, creating a need for efficient energy storage solutions to ensure a consistent power supply. Various technologies exist to address this challenge, each with its own strengths and weaknesses. Understanding these differences is crucial for optimizing grid stability and maximizing the utilization of renewable energy resources.
Classification of Renewable Energy Storage Technologies
Renewable energy storage technologies can be broadly categorized based on their storage mechanism. These categories offer diverse solutions, each suitable for different applications and scales. The choice of technology often depends on factors such as cost, energy density, lifespan, and environmental impact.
Pumped Hydro Storage
Pumped hydro storage (PHS) is a mature and widely used technology. It involves pumping water uphill to a reservoir during periods of low energy demand and then releasing it to generate electricity during peak demand. This process utilizes the potential energy of water. Large-scale PHS plants are typically located in mountainous or hilly regions where significant elevation differences exist.
A notable example is the Bath County Pumped Storage Station in Virginia, USA.Advantages of PHS include its high energy capacity, long lifespan, and relatively low operating costs. However, PHS requires significant land and water resources, making it geographically limited and potentially environmentally impactful due to habitat disruption and water usage.
Battery Storage
Battery storage is a rapidly developing sector offering various chemistries. Lithium-ion batteries currently dominate the market due to their high energy density and relatively long lifespan. However, other chemistries like flow batteries are gaining traction for large-scale applications. Flow batteries, unlike lithium-ion, can independently scale energy capacity and power output, offering flexibility in design and deployment.Advantages of battery storage include their modularity, fast response times, and high efficiency.
However, the cost of batteries, particularly lithium-ion, remains a significant barrier to widespread adoption. Furthermore, concerns exist regarding the environmental impact of battery manufacturing and disposal, particularly concerning the sourcing and recycling of materials like lithium and cobalt.
Compressed Air Energy Storage
Compressed air energy storage (CAES) involves compressing air during off-peak hours and then releasing it to drive turbines and generate electricity when needed. This technology can be particularly useful for integrating intermittent renewable energy sources into the grid. CAES systems can be designed to store energy for extended periods, offering a solution for managing long-term energy imbalances. A noteworthy example is the McIntosh CAES plant in Alabama, USA, which has been operational since the 1990s.Advantages of CAES include its scalability and relatively low environmental impact compared to some other storage technologies.
However, CAES systems are often less efficient than other storage methods due to energy losses during compression and expansion. The cost of construction and maintenance can also be substantial.
Thermal Energy Storage
Thermal energy storage (TES) involves storing energy in the form of heat or cold. This can be achieved using various materials, such as molten salts, water, or rocks. TES is particularly well-suited for applications such as solar thermal power plants and district heating systems. By storing excess heat during sunny periods, TES enables continuous power generation even when solar irradiance is low.Advantages of TES include its potential for large-scale energy storage and its ability to provide long-duration storage.
Effective renewable energy storage solutions are crucial for maximizing the benefits of intermittent sources like wind power. Understanding the advantages of harnessing wind energy is key, and a great resource to explore this is the article on Benefits of wind power for rural communities , which highlights its potential for rural electrification. Ultimately, advancements in storage will allow for a more reliable and consistent supply of clean energy from wind farms, further enhancing the positive impacts on rural areas.
However, the efficiency of TES systems can be limited by heat losses during storage and retrieval. The high capital costs associated with constructing and maintaining large-scale TES facilities can also be a barrier to adoption.
Storage Method | Energy Density (kWh/m³) | Lifespan (years) | Cost ($/kWh) | Environmental Impact |
---|---|---|---|---|
Pumped Hydro | 100-500 | 50+ | 100-300 | Moderate (land use, water consumption) |
Lithium-ion Battery | 250-500 | 10-20 | 200-500 | Moderate (material sourcing, recycling) |
Flow Battery | 10-50 | 20+ | 300-600 | Low to moderate (depending on electrolyte) |
Compressed Air | 10-100 | 20+ | 200-500 | Low |
Thermal (Molten Salt) | 50-200 | 20+ | 200-400 | Low |
Technological Advancements in Storage Solutions
The field of renewable energy storage is experiencing rapid evolution, driven by the increasing need for reliable and efficient energy management. Recent breakthroughs are significantly improving the performance, cost-effectiveness, and lifespan of various storage technologies, paving the way for a more sustainable energy future. These advancements are crucial for addressing the intermittency of renewable sources like solar and wind power, ensuring a consistent energy supply.Recent breakthroughs and innovations are transforming the landscape of renewable energy storage.
Improved battery chemistries, advancements in pumped hydro storage, and the emergence of novel technologies like flow batteries and compressed air energy storage are all contributing to a more robust and efficient energy storage infrastructure. These advancements are not only increasing the capacity and duration of energy storage but also enhancing its overall efficiency and reducing its environmental impact.
Advancements in Battery Technologies
Lithium-ion batteries currently dominate the renewable energy storage market. However, ongoing research focuses on improving their energy density, cycle life, and safety. Significant progress has been made with solid-state batteries, which offer enhanced safety and potentially higher energy density compared to their liquid electrolyte counterparts. Researchers are also exploring alternative battery chemistries, such as sodium-ion and zinc-ion batteries, which offer potential cost advantages and improved sustainability.
For example, solid-state batteries are expected to increase the range of electric vehicles significantly, mirroring the advancements seen in renewable energy storage applications. The development of high-nickel cathode materials has also increased the energy density of lithium-ion batteries, leading to smaller and more powerful storage solutions.
Development Timeline of Key Renewable Energy Storage Technologies
The following flowchart illustrates the key milestones in the development of major renewable energy storage technologies:(Imagine a flowchart here. It would start with early experimental work on various technologies in the late 19th and early 20th centuries. Then branches would show the development of pumped hydro storage (PHS) starting in the early 20th century with significant growth in the mid-20th century.
Another branch would show the development of lead-acid batteries, also starting in the late 19th century, with gradual improvements over time. A third branch would illustrate the rapid development of lithium-ion batteries starting in the 1970s and accelerating in the 1990s and 2000s. Finally, newer technologies like flow batteries and compressed air energy storage would be shown emerging more recently, in the late 20th and early 21st centuries, with ongoing development.)
Limitations and Potential Solutions for Existing Storage Technologies
Current renewable energy storage technologies face several limitations. Lithium-ion batteries, while dominant, suffer from limited cycle life, high initial costs, and concerns about the sourcing and recycling of materials. Pumped hydro storage, while mature and reliable, requires geographically specific sites and can have significant environmental impacts. Flow batteries, although promising, currently have lower energy densities compared to lithium-ion batteries and can be expensive.Potential solutions include developing more sustainable and cost-effective battery chemistries, improving battery management systems to extend cycle life, and exploring hybrid storage systems that combine the advantages of different technologies.
Efficient renewable energy storage solutions are crucial for a sustainable future, particularly in powering transportation. The widespread adoption of electric vehicles hinges on reliable access to clean energy sources, a topic explored in detail at Green energy for electric vehicles. Therefore, advancements in renewable energy storage are directly linked to the success of green initiatives in the automotive sector.
For pumped hydro, innovative designs and improved siting strategies can mitigate environmental impacts. Further research and development are crucial to overcome the limitations of existing technologies and make renewable energy storage more affordable, efficient, and environmentally benign. For example, research into closed-loop pumped hydro systems reduces water consumption and environmental impact. Similarly, advancements in battery recycling technologies will address the issue of material sourcing and disposal.
Economic and Policy Aspects of Renewable Energy Storage
The economic viability and widespread adoption of renewable energy storage are intrinsically linked to both technological advancements and supportive policy frameworks. A comprehensive understanding of the levelized cost of electricity (LCOE), government incentives, and private investment is crucial for forecasting the future of this critical sector. This section explores these interconnected aspects, providing insights into the financial and regulatory landscape shaping the renewable energy storage market.
Levelized Cost of Electricity (LCOE) for Various Storage Technologies
The levelized cost of electricity (LCOE) provides a standardized metric for comparing the cost-effectiveness of different energy sources, including renewable energy storage technologies. LCOE considers the total cost of building and operating a power plant over its lifetime, divided by the total energy produced. Different storage technologies exhibit varying LCOEs, influenced by factors like capital costs, operational expenses, lifespan, and efficiency.
For example, pumped hydro storage, due to its established infrastructure and relatively low operational costs, generally boasts a lower LCOE than emerging technologies like lithium-ion batteries. However, the LCOE for lithium-ion batteries is rapidly decreasing due to economies of scale and technological improvements, making them increasingly competitive in certain applications. A comparative analysis reveals that while pumped hydro storage currently maintains a cost advantage in large-scale deployments, battery storage solutions are becoming more economically viable for smaller-scale and grid-balancing applications.
Further research and development, coupled with continued cost reductions, are expected to further narrow the gap between these and other technologies, such as compressed air energy storage (CAES) and flow batteries.
Impact of Government Policies and Subsidies on Renewable Energy Storage Adoption
Government policies and subsidies play a pivotal role in accelerating the adoption of renewable energy storage. Direct financial incentives, such as tax credits, grants, and rebates, can significantly reduce the upfront capital costs associated with storage deployment. Regulatory frameworks, including renewable portfolio standards (RPS) and mandates for grid modernization, can also create a favorable market environment for storage technologies by requiring utilities to integrate renewable energy sources, including storage, into their electricity grids.
For instance, many countries are implementing policies that prioritize the integration of renewable energy and storage solutions, creating demand and encouraging investment. Conversely, the absence of supportive policies or the presence of regulatory barriers can hinder the growth of the renewable energy storage sector. The success of policies such as feed-in tariffs and tax breaks for renewable energy sources in incentivizing their deployment serves as a precedent for how similar policies can stimulate renewable energy storage adoption.
Role of Private Investment and Venture Capital in Driving Innovation
Private investment and venture capital are crucial drivers of innovation in the renewable energy storage sector. Significant funding from private investors and venture capitalists has fueled research and development efforts, leading to advancements in battery chemistry, energy management systems, and other key technologies. These investments often target promising start-ups and established companies developing cutting-edge storage solutions. The expectation of high returns on investment in this rapidly growing sector attracts considerable capital, supporting the development of new technologies and the expansion of existing ones.
Successful examples of venture capital-backed companies achieving commercial success in renewable energy storage illustrate the potential for private investment to accelerate innovation and market penetration. The competitive landscape further encourages continuous improvement and cost reduction, creating a virtuous cycle of innovation and investment.
Integration of Renewable Energy Storage into the Grid
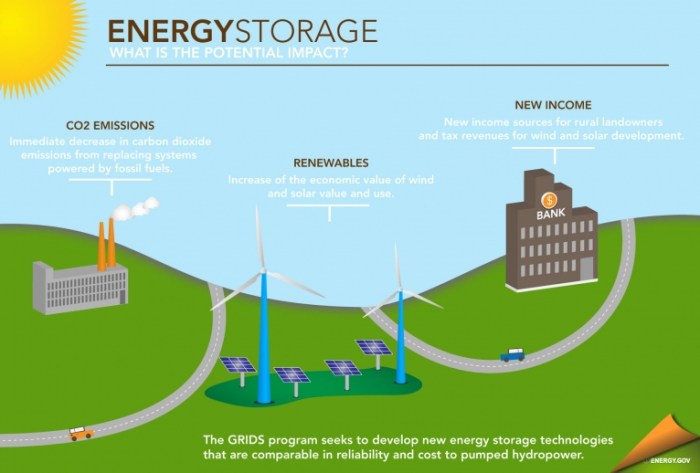
Source: ensightenergyllc.com
Integrating large-scale renewable energy storage systems into existing power grids presents both significant challenges and exciting opportunities. The intermittent nature of renewable sources like solar and wind necessitates effective storage solutions to ensure a consistent and reliable power supply. This integration requires careful planning and technological advancements to manage the complexities of grid operation and maintain stability.The successful integration of renewable energy storage hinges on addressing several key challenges.
One major hurdle is the need for robust grid infrastructure capable of handling the influx of energy from diverse sources, including intermittent renewables and storage systems. Existing grids were often designed for unidirectional power flow from centralized generation, a paradigm shift is needed to accommodate bidirectional flows associated with storage. Furthermore, effective control systems are crucial to manage the dynamic interactions between renewable energy sources, storage, and grid demand, preventing instability and ensuring seamless power delivery.
Finally, the economic viability of large-scale storage deployment, including capital costs, operational expenses, and potential revenue streams, needs careful consideration to incentivize widespread adoption.
Effective renewable energy storage solutions are crucial for maximizing the benefits of intermittent sources like solar and wind. The successful implementation of these storage systems is directly linked to the growth of renewable energy projects, such as those detailed in this overview of Renewable energy projects in the US. Therefore, continued investment in both areas is vital for a sustainable energy future.
Challenges of Integrating Large-Scale Renewable Energy Storage Systems, Renewable energy storage solutions
Integrating large-scale renewable energy storage systems into existing power grids presents a number of significant challenges. These include the need for upgraded grid infrastructure to handle the increased capacity and bidirectional power flows, the development of advanced control systems to manage the dynamic interplay between renewable energy sources and storage, and the need for cost-effective storage solutions that can compete with traditional generation methods.
For example, the California Independent System Operator (CAISO) has faced challenges integrating large amounts of solar power, leading to the need for flexible resources, including energy storage, to manage fluctuations in supply and demand. Addressing these challenges is critical to ensuring the reliable and efficient integration of renewable energy into the grid.
Opportunities Presented by Widespread Renewable Energy Storage Adoption
Widespread adoption of renewable energy storage offers several significant opportunities for improving grid stability and reliability. The ability to store excess renewable energy during periods of high generation and release it during periods of peak demand can significantly reduce reliance on fossil fuel-based peaker plants, thereby decreasing greenhouse gas emissions and improving air quality. Moreover, storage can help mitigate the intermittency of renewable sources, providing a more consistent and reliable power supply.
This improved reliability enhances grid resilience to disruptions, such as extreme weather events, and reduces the risk of blackouts. The integration of storage also opens the door for advancements in grid management, enabling more efficient and cost-effective grid operation. For instance, the integration of pumped hydro storage in Norway has significantly enhanced the country’s grid stability and reliability, while reducing reliance on fossil fuel-based power plants.
Impact of Widespread Renewable Energy Storage Adoption on Grid Stability and Reliability: A Scenario
Consider a scenario where a significant portion of a region’s electricity generation is powered by solar and wind energy, supplemented by a widespread network of energy storage systems. During peak sunlight hours, solar farms generate excess energy, which is stored in batteries and pumped hydro storage facilities. As the sun sets and wind speeds decrease, the stored energy is seamlessly released into the grid, ensuring a continuous power supply.
This prevents the sudden dips in electricity supply that can occur with solely relying on intermittent renewables, reducing the frequency and severity of load shedding and blackouts. The grid becomes more resilient to unexpected events, like sudden changes in weather or equipment malfunctions, due to the buffer provided by the stored energy. This scenario highlights the transformative potential of renewable energy storage in enhancing grid stability and reliability.
Benefits of Smart Grid Technologies in Optimizing Renewable Energy Storage Systems
Smart grid technologies play a crucial role in optimizing the operation and control of renewable energy storage systems. These technologies utilize advanced sensors, communication networks, and data analytics to monitor grid conditions in real-time, predict energy demand, and optimize the dispatch of energy from renewable sources and storage systems. This allows for a more efficient and responsive grid operation, maximizing the utilization of renewable energy and minimizing reliance on fossil fuels.
The diagram illustrates the interaction between renewable energy sources (solar and wind), energy storage (battery), and a smart grid. Renewable sources feed energy into the storage system, which in turn releases energy to the grid based on real-time demand and grid conditions monitored by the smart grid’s sensors and communication networks. The smart grid uses data analytics to optimize energy flow, maximizing the utilization of renewable energy and minimizing grid instability.
This optimized control minimizes reliance on traditional power plants and ensures a reliable and stable electricity supply.
Environmental Impact and Sustainability Considerations
The environmental impact of renewable energy storage is a crucial factor in determining its overall sustainability. While renewable energy sources themselves are environmentally friendly, the technologies used to store their energy can have significant environmental consequences throughout their lifecycle, from material extraction and manufacturing to operation and eventual disposal. A comprehensive assessment of these impacts is necessary to ensure the responsible and sustainable deployment of renewable energy storage solutions.The environmental footprint of renewable energy storage varies considerably depending on the technology employed.
Different storage methods have unique challenges related to material sourcing, manufacturing processes, operational energy consumption, and end-of-life management. Comparing these impacts across the lifecycle allows for a more informed evaluation of each technology’s overall sustainability.
Manufacturing Impacts of Different Renewable Energy Storage Technologies
Manufacturing processes for various renewable energy storage technologies consume significant energy and resources, generating greenhouse gas emissions and potentially polluting air and water. For example, lithium-ion batteries, a dominant technology in energy storage, require the mining of lithium, cobalt, and nickel, processes that can cause habitat destruction, water pollution, and generate substantial carbon emissions. Pumped hydro storage, while utilizing existing infrastructure, still involves construction activities with associated environmental impacts like habitat disruption and land use changes.
Conversely, technologies like compressed air energy storage (CAES) may have lower material demands but could require substantial land usage for air storage caverns. The environmental burdens associated with manufacturing vary greatly depending on the specific technology and the sourcing and processing of raw materials.
Lifecycle Carbon Footprint Comparison of Renewable Energy Storage Options
A comprehensive lifecycle assessment (LCA) considers all stages, from raw material extraction to end-of-life disposal, allowing for a fair comparison of the carbon footprint of different renewable energy storage options. Studies show that lithium-ion batteries generally have a higher carbon footprint than pumped hydro storage, particularly due to the energy-intensive manufacturing processes and the embodied carbon in their materials. However, advancements in battery chemistry and manufacturing techniques are continuously striving to reduce this footprint.
Other technologies, like flow batteries and thermal energy storage, present varying carbon footprints depending on the specific materials and manufacturing processes involved. The overall goal is to minimize the total lifecycle carbon emissions associated with each storage solution. For example, a recent study showed that the lifecycle carbon emissions of a lithium-ion battery system for a solar power plant in Arizona were estimated to be approximately 100-200 gCO2e/kWh over its 15-year lifespan, while a pumped hydro storage system in the same location had a significantly lower footprint, estimated to be around 10-20 gCO2e/kWh over a longer lifespan.
Sustainable Practices for Minimizing Environmental Impact
Several strategies can be implemented to mitigate the environmental impact of renewable energy storage. These include the development of more sustainable manufacturing processes with reduced energy consumption and waste generation, the use of recycled materials, and the exploration of alternative materials with lower environmental impacts. Improving battery lifespan and recyclability is also crucial. Responsible sourcing of raw materials, emphasizing ethical and environmentally sound mining practices, is essential.
Furthermore, efficient end-of-life management strategies, including recycling and reuse programs, are vital for minimizing the environmental burden of discarded storage systems. The development of standardized and robust recycling infrastructure for various storage technologies is critical to achieving circular economy principles and reducing waste. For instance, companies are actively developing closed-loop recycling processes for lithium-ion batteries to recover valuable materials and minimize waste.
These efforts are crucial for creating a more sustainable future for renewable energy storage.
Future Trends and Research Directions in Renewable Energy Storage
The field of renewable energy storage is rapidly evolving, driven by the increasing need for reliable and efficient energy solutions. Future advancements will focus on improving existing technologies while exploring novel approaches to overcome current limitations in cost, efficiency, and scalability. This necessitates a multi-pronged research effort encompassing materials science, engineering, and economic modeling.
Nanotechnology and Advanced Materials in Renewable Energy Storage
The application of nanotechnology offers significant potential for enhancing the performance and lifespan of renewable energy storage systems. Nanomaterials, due to their unique properties like high surface area and tunable electronic characteristics, can improve energy density, power density, and cycle life of batteries, supercapacitors, and other storage devices. For instance, the use of graphene-based materials in lithium-ion batteries has shown promising results in increasing energy density and charging rates.
Renewable energy storage solutions are crucial for maximizing the benefits of intermittent sources like solar and wind. The widespread adoption of these solutions is significantly contributing to economic expansion, as detailed in this insightful article: How green energy is driving economic growth. Ultimately, advancements in storage technology are key to unlocking the full potential of renewable energy and further stimulating economic growth.
Similarly, the development of advanced electrode materials using nanowires or nanotubes is leading to batteries with improved performance and longevity. Research is also exploring the use of nanomaterials to enhance the efficiency of other energy storage methods, such as fuel cells and flow batteries.
Novel Storage Technologies: Gravity-Based and Flywheel Energy Storage
Beyond advancements in established technologies, novel storage solutions are gaining traction. Gravity-based energy storage (GBES) systems utilize the potential energy of elevated masses to store energy. In practice, this involves lifting heavy weights during periods of surplus energy generation and lowering them to release energy when demand is high. This technology offers a long lifespan and high efficiency, with minimal environmental impact.
For example, Energy Vault is a company actively deploying GBES systems using modular concrete blocks. Flywheel energy storage, another promising technology, stores energy in the kinetic energy of a rotating flywheel. Advances in materials science, particularly the development of high-strength, lightweight materials, are improving the energy density and efficiency of flywheel systems. These systems offer fast response times and are suitable for grid-scale applications requiring rapid frequency regulation.
Companies like Beacon Power have demonstrated the viability of flywheel energy storage in real-world applications.
Improved Grid Integration Strategies
Future research will focus on optimizing the integration of diverse energy storage technologies into the electrical grid. This involves developing sophisticated control algorithms and smart grid technologies that can effectively manage the flow of energy from renewable sources and storage systems, ensuring grid stability and reliability. Real-time energy forecasting models, combined with advanced grid management systems, will play a crucial role in optimizing energy storage deployment and operation.
This will require further development of sophisticated algorithms and improved communication infrastructure within the grid.
Closure
In conclusion, the future of renewable energy hinges significantly on the continued development and deployment of effective storage solutions. Addressing the challenges of cost, efficiency, environmental impact, and grid integration is crucial for widespread adoption. Ongoing innovation, supportive policies, and strategic investment will be essential to unlock the full potential of renewable energy and pave the way for a cleaner, more sustainable energy future.
The journey towards a truly sustainable energy system requires a collaborative effort, encompassing technological advancements, economic viability, and responsible environmental stewardship.
Expert Answers
What are the safety concerns associated with different energy storage technologies?
Safety concerns vary by technology. Lithium-ion batteries, for example, pose fire risks if not properly managed. Pumped hydro storage has potential risks associated with dam failure. Thorough risk assessments and safety protocols are essential for all storage technologies.
How long does it take to build a large-scale renewable energy storage facility?
The construction time varies greatly depending on the technology and scale of the project. Pumped hydro projects, for example, can take several years, while smaller-scale battery installations may be completed in months.
What is the role of blockchain technology in renewable energy storage?
Blockchain can enhance transparency and traceability in renewable energy trading and storage management, improving efficiency and accountability in the energy market.
What are the geopolitical implications of renewable energy storage technology?
Control over critical minerals used in battery technologies, for instance, can create geopolitical dependencies. The development and deployment of diverse storage technologies can mitigate these risks.