Energy Management Systems A Comprehensive Overview
Energy Management Systems (EMS) represent a crucial advancement in optimizing energy consumption across diverse sectors. From sprawling industrial complexes to individual buildings, EMS leverage sophisticated technologies to monitor, analyze, and control energy usage, leading to significant cost savings and environmental benefits. This exploration delves into the core functionalities, applications, and future trends of EMS, providing a detailed understanding of their transformative potential.
This overview examines the historical development of EMS, detailing the evolution from rudimentary systems to the sophisticated, interconnected networks we see today. We will explore various types of EMS, including those tailored for buildings, industrial settings, and smart grids, highlighting their unique architectures and functionalities. The economic and environmental advantages will be thoroughly discussed, supported by real-world examples and comparative analyses of different technologies.
Introduction to Energy Management Systems (EMS)
Energy Management Systems (EMS) are sophisticated technological solutions designed to monitor, analyze, and optimize energy consumption within a defined area or system. Their core functionalities encompass real-time data acquisition from various energy-consuming devices, data analysis to identify trends and inefficiencies, and the implementation of control strategies to reduce energy waste and improve overall energy efficiency. EMS offer a comprehensive approach to energy management, providing valuable insights and actionable recommendations for reducing operational costs and environmental impact.EMS have evolved significantly over time.
Early systems were primarily focused on simple monitoring and alarming, using rudimentary hardware and software. The advent of more powerful computing capabilities and advanced communication technologies, such as the internet and more recently, the Internet of Things (IoT), has driven a rapid expansion in the functionalities and capabilities of EMS. Modern EMS leverage sophisticated algorithms and machine learning to predict energy demand, optimize energy distribution, and automate control processes, resulting in significant improvements in energy efficiency and cost savings.
Types of Energy Management Systems
Different types of EMS cater to specific needs and scales of operation. Building EMS focus on optimizing energy use within individual buildings, encompassing HVAC systems, lighting, and other equipment. Industrial EMS manage energy consumption in large-scale industrial processes, often integrating with production control systems to optimize energy usage alongside production efficiency. Smart Grid EMS are used to manage and control the flow of electricity across an entire power grid, balancing supply and demand in real-time to ensure reliable and efficient power distribution.
Each type employs tailored strategies and technologies to address the unique energy management challenges of its respective environment.
Effective Energy Management Systems are crucial for modern buildings. A key component of a comprehensive strategy often involves optimizing heating and cooling, which is where a device like a Smart Thermostat becomes invaluable. These smart thermostats allow for automated adjustments based on occupancy and weather, ultimately leading to significant energy savings and improved overall efficiency within the Energy Management System.
Key Components of EMS Architecture
A typical EMS architecture consists of several key components working in concert. These include sensors and meters that collect data on energy consumption from various sources, communication networks that transmit this data to a central control system, a data processing and analysis unit that interprets the data and identifies optimization opportunities, and a control system that implements strategies to adjust energy usage based on the analysis.
A user interface provides access to the system’s data and functionalities, allowing users to monitor performance, configure settings, and generate reports. Sophisticated EMS often integrate advanced analytics capabilities, such as predictive modeling and machine learning, to further enhance energy optimization. For instance, a building EMS might use weather forecasting data to preemptively adjust HVAC settings, minimizing energy waste.
Similarly, an industrial EMS might use production scheduling data to optimize energy usage during peak production periods.
Benefits and Applications of EMS
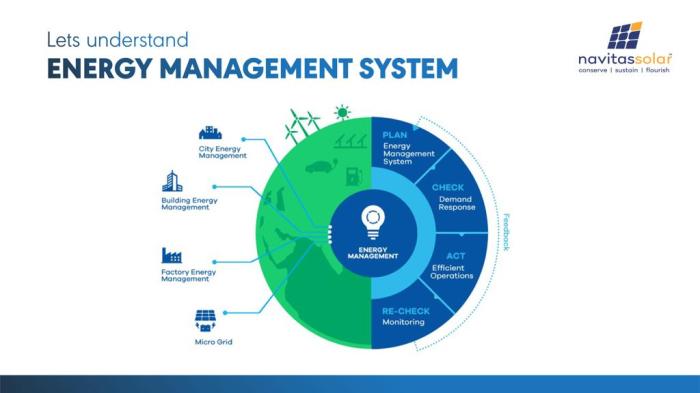
Source: navitassolar.in
Energy Management Systems (EMS) offer a compelling blend of economic and environmental advantages, leading to significant improvements in operational efficiency and sustainability across diverse sectors. Implementing an EMS is not simply about reducing energy bills; it’s about optimizing resource utilization, minimizing environmental impact, and enhancing overall operational performance. This section will delve into the specific benefits and showcase successful applications of EMS in various industries.
Economic Benefits of EMS Implementation
The financial incentives for adopting an EMS are substantial. Cost savings are realized through reduced energy consumption, lower operational expenses, and improved asset management. A well-designed EMS can pinpoint energy waste, leading to targeted interventions that directly translate into lower utility bills. Furthermore, the optimized efficiency can extend the lifespan of equipment, reducing the need for premature replacements.
The return on investment (ROI) varies depending on the complexity of the system and the specific energy consumption patterns of the facility, but studies consistently show significant positive returns within a relatively short timeframe. For example, a manufacturing plant might see a 15-20% reduction in energy costs within the first year, leading to a rapid ROI. This cost reduction can then be reinvested into other business operations or used to offset other expenses.
Environmental Benefits of EMS
Beyond the economic advantages, EMS contribute significantly to environmental sustainability. By reducing energy consumption, EMS directly lower greenhouse gas emissions, contributing to a smaller carbon footprint. This aligns with global efforts to mitigate climate change and meet sustainability targets. Moreover, optimized energy use often translates to less waste generation and reduced reliance on fossil fuels, further benefiting the environment.
For instance, a commercial building equipped with an EMS might see a 30% reduction in its carbon emissions, a substantial contribution to a greener future. This reduction is not only beneficial for the environment but can also enhance the building’s reputation and attract environmentally conscious tenants or customers.
Successful EMS Implementations Across Sectors
EMS have proven their effectiveness across various sectors. In healthcare, EMS are used to monitor and control energy consumption in hospitals, optimizing HVAC systems and medical equipment to reduce energy waste while ensuring patient comfort and safety. In manufacturing, EMS optimize production processes, minimizing energy use during peak demand periods and improving overall production efficiency. Commercial buildings benefit from EMS through optimized lighting, HVAC, and security systems, leading to lower operational costs and improved tenant satisfaction.
For example, a large hospital system might utilize an EMS to centrally manage energy usage across multiple facilities, leading to significant savings and a reduced environmental impact across the entire system. Similarly, a manufacturing plant can use an EMS to monitor and control energy use during different production phases, leading to substantial cost reductions and reduced emissions.
Comparison of EMS Technologies
EMS Technology | Advantages | Disadvantages | Suitable Applications |
---|---|---|---|
Building Automation Systems (BAS) | Comprehensive control, integration with other building systems, scalability | High initial investment, complexity of implementation, requires specialized expertise | Large commercial buildings, industrial facilities |
Supervisory Control and Data Acquisition (SCADA) | Real-time monitoring and control, data visualization, remote access | Can be complex to configure and maintain, requires specialized training | Industrial processes, power grids, water treatment plants |
Energy Information Systems (EIS) | Data analysis and reporting, identification of energy waste, trend analysis | Limited control capabilities, relies on data accuracy from other systems | Energy audits, performance benchmarking, compliance reporting |
Smart Meters | Accurate energy consumption data, real-time monitoring, remote meter reading | Limited control capabilities, requires robust communication infrastructure | Residential, commercial, and industrial buildings |
Key Features and Technologies in EMS
Energy Management Systems (EMS) rely on a sophisticated interplay of hardware and software to achieve efficient energy control. Their core functionality centers around acquiring data from various sources, monitoring energy consumption patterns, and implementing control strategies to optimize energy use and reduce waste. This involves a complex communication network and advanced analytical tools.
Core Functionalities of an EMS
An EMS’s core functionalities can be broadly categorized into data acquisition, monitoring, and control. Data acquisition involves gathering real-time information on energy consumption from diverse sources within a building or facility. This data is then monitored to identify trends, anomalies, and areas for improvement. Finally, control mechanisms are implemented based on this analysis to adjust energy usage, optimizing efficiency and minimizing costs.
For example, an EMS might automatically adjust HVAC settings based on occupancy levels or external weather conditions, resulting in significant energy savings. The system might also generate alerts for potential equipment malfunctions, enabling proactive maintenance.
Communication Protocols in EMS
Effective communication between various components of an EMS is crucial. Several communication protocols facilitate this data exchange. Modbus is a widely used serial communication protocol known for its simplicity and robustness, frequently employed in industrial automation and building control systems. BACnet (Building Automation and Control Networks) is another popular protocol specifically designed for building automation, supporting a wide range of devices and functionalities.
OPC UA (Open Platform Communications Unified Architecture) is a more modern, interoperable protocol offering enhanced security and scalability, increasingly favored for complex, heterogeneous systems. The choice of protocol depends on factors such as the scale of the system, the types of devices involved, and the desired level of interoperability.
Effective Energy Management Systems are crucial for modern buildings. Optimizing energy consumption often involves smart technologies that adapt to environmental conditions, such as integrating automated systems for climate control. For instance, the strategic use of Automated Blinds can significantly reduce reliance on artificial lighting and air conditioning, ultimately leading to substantial energy savings within a comprehensive Energy Management System.
The Role of Sensors, Actuators, and Data Analytics in Modern EMS
Sensors form the foundation of data acquisition in an EMS. They continuously monitor various parameters such as temperature, humidity, power consumption, and occupancy levels. This data is then transmitted to the EMS via communication protocols. Actuators, on the other hand, are the control elements that respond to commands from the EMS to adjust equipment settings. For instance, a thermostat acting as an actuator might receive a command from the EMS to lower the temperature in a room based on occupancy data.
Data analytics plays a critical role in processing the vast amounts of data gathered by sensors, identifying patterns, and generating insights for improved energy management. Advanced analytics techniques like machine learning can predict energy consumption, optimize control strategies, and proactively identify potential issues.
Data Flow within an EMS
The following flowchart illustrates a simplified representation of data flow within a typical EMS:[Imagine a flowchart here. The flowchart would start with “Sensors (Temperature, Power, Occupancy, etc.)” pointing to “Data Acquisition System”. The Data Acquisition System would then point to “Communication Network (Modbus, BACnet, OPC UA)”. The Communication Network would point to “EMS Central Processing Unit”. The EMS CPU would point to two separate branches: “Data Analytics & Visualization” and “Control System (Actuators)”.
The “Data Analytics & Visualization” branch would loop back to the “EMS Central Processing Unit” indicating feedback. The “Control System (Actuators)” branch would point to “HVAC, Lighting, etc.” Finally, “HVAC, Lighting, etc.” would loop back to “Sensors”, completing the cycle.]
Implementing and Managing an EMS
Implementing an Energy Management System (EMS) requires a strategic approach encompassing planning, installation, and ongoing optimization. A successful EMS implementation leads to significant energy savings, improved operational efficiency, and reduced environmental impact. This section details the steps involved, best practices, potential challenges, and crucial considerations for selecting the right EMS solution.
Step-by-Step EMS Implementation in a Typical Building
Implementing an EMS in a building is a multi-stage process. It begins with a thorough assessment of the building’s energy consumption patterns and progresses through design, installation, commissioning, and ongoing monitoring and optimization. A phased approach often proves most effective, allowing for incremental improvements and adjustments along the way.
- Needs Assessment and Planning: This initial phase involves a comprehensive energy audit to identify areas of high energy consumption and potential for improvement. This audit should include detailed analysis of HVAC systems, lighting, and other major energy-consuming equipment. The assessment should define project goals, budget, and timeline.
- System Design and Selection: Based on the needs assessment, the appropriate EMS solution is selected. This involves choosing hardware (sensors, controllers, gateways) and software (monitoring platform, data analytics tools) tailored to the building’s specific requirements. Integration with existing building automation systems (BAS) should be considered.
- Installation and Commissioning: This stage involves the physical installation of sensors, controllers, and other hardware components throughout the building. Commissioning ensures the system is correctly installed and functions as designed, involving rigorous testing and validation of all components and their interactions.
- Training and User Acceptance Testing: Building occupants and staff require training on how to use and interact with the EMS. User acceptance testing (UAT) allows for feedback and adjustments before full deployment.
- Ongoing Monitoring and Optimization: Continuous monitoring of energy consumption data is crucial. The EMS should provide real-time insights into energy usage, allowing for proactive adjustments and optimization strategies to maximize efficiency and minimize waste.
Best Practices for Optimizing EMS Performance
Optimizing EMS performance requires a proactive and data-driven approach. Regular maintenance, software updates, and continuous monitoring are essential. Effective communication and collaboration among building management, engineers, and occupants also play a vital role.
- Regular calibration and maintenance of sensors and other hardware components to ensure accuracy and reliability.
- Implementation of automated control strategies based on real-time data analysis, such as optimizing HVAC schedules based on occupancy patterns.
- Utilizing advanced analytics and machine learning algorithms to identify and address energy waste and improve predictive maintenance.
- Establishing clear key performance indicators (KPIs) to track progress and measure the effectiveness of the EMS.
- Regular review and updates of the EMS software to incorporate new features and improvements.
Challenges Associated with EMS Implementation and Maintenance
EMS implementation can present several challenges. These include integration complexities, initial investment costs, data security concerns, and the need for skilled personnel to manage and maintain the system.
- Integration Complexity: Integrating an EMS with existing building systems can be challenging, requiring careful planning and coordination.
- High Initial Investment Costs: The initial investment in hardware, software, and installation can be substantial.
- Data Security Concerns: Protecting sensitive building data from unauthorized access is crucial.
- Need for Skilled Personnel: Managing and maintaining an EMS requires skilled personnel with expertise in building automation and energy management.
Checklist for Selecting an Appropriate EMS Solution
Choosing the right EMS solution is crucial for successful implementation. The selection process should involve a thorough evaluation of various vendors and their offerings. Factors to consider include scalability, integration capabilities, user-friendliness, and long-term support.
Factor | Considerations |
---|---|
Scalability | Can the system accommodate future expansion and changes in building usage? |
Integration Capabilities | Does the system integrate seamlessly with existing building systems (HVAC, lighting, etc.)? |
User-Friendliness | Is the system easy to use and understand for building operators and occupants? |
Data Analytics and Reporting | Does the system provide comprehensive data analytics and reporting capabilities? |
Vendor Support and Maintenance | Does the vendor provide reliable support and maintenance services? |
Cost | What is the total cost of ownership (TCO), including hardware, software, installation, and ongoing maintenance? |
Future Trends in Energy Management Systems
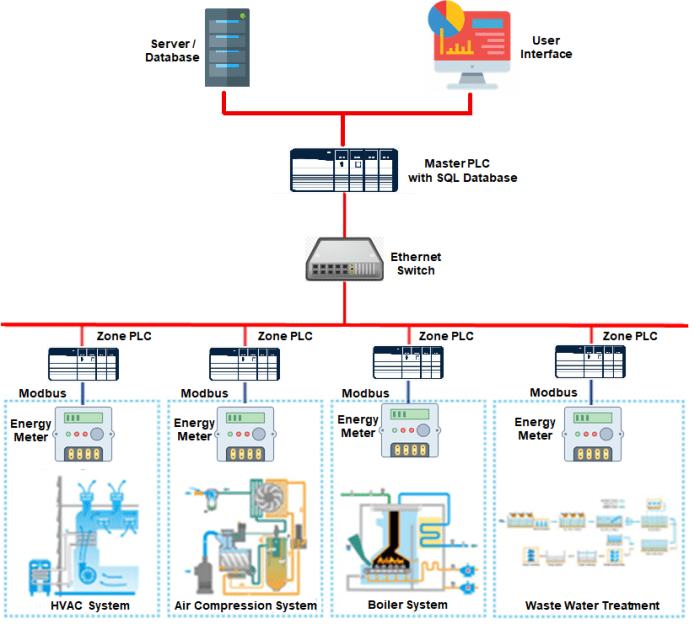
Source: stability.co
Energy Management Systems (EMS) are poised for significant advancements driven by rapid technological progress. The integration of emerging technologies is not just enhancing existing capabilities but fundamentally reshaping the landscape of energy management, paving the way for more efficient, sustainable, and intelligent systems. This section explores the key trends shaping the future of EMS.The convergence of artificial intelligence (AI), machine learning (ML), and the Internet of Things (IoT) is revolutionizing energy management.
These technologies are enabling predictive analytics, real-time optimization, and proactive maintenance, leading to substantial cost savings and improved energy efficiency. Moreover, the increasing availability of big data and advanced computing power fuels the development of more sophisticated and adaptable EMS solutions.
Effective Energy Management Systems are crucial for modern businesses, impacting both operational costs and environmental responsibility. A key aspect often overlooked is the building’s design itself; consider the impact of features like natural light and efficient HVAC systems, as explored in detail through resources on Office Interior Design. Ultimately, integrating thoughtful design with smart energy management technologies leads to significant long-term savings and sustainability.
The Impact of AI and Machine Learning on EMS
AI and ML algorithms are transforming how energy is consumed and managed. Machine learning models can analyze vast amounts of energy consumption data to identify patterns, predict future demand, and optimize energy distribution in real-time. This predictive capability allows for proactive adjustments to energy production and consumption, minimizing waste and maximizing efficiency. For example, AI can predict peak demand hours with greater accuracy, enabling utilities to adjust generation schedules accordingly and avoid costly overproduction or energy shortages.
Furthermore, ML algorithms can identify anomalies in energy consumption, flagging potential equipment malfunctions or energy theft, allowing for timely intervention and preventative maintenance. This proactive approach minimizes downtime and reduces repair costs.
The Role of the Internet of Things (IoT) in the Future of EMS
The Internet of Things (IoT) is crucial in providing the granular data needed for effective AI and ML-driven EMS. By connecting various energy-consuming devices and systems—from smart meters and HVAC systems to solar panels and electric vehicle chargers—the IoT creates a network of interconnected sensors that continuously monitor energy usage. This real-time data stream allows for precise energy consumption tracking, enabling more accurate demand forecasting and optimized resource allocation.
Imagine a smart building where IoT sensors monitor energy usage in individual rooms, automatically adjusting lighting and climate control based on occupancy and external conditions. This level of granular control leads to significant energy savings and improved comfort. The IoT also facilitates remote monitoring and control, allowing energy managers to oversee and manage multiple sites from a central location.
Effective Energy Management Systems are crucial for modern homes, aiming to optimize energy consumption and reduce costs. A key component of achieving this efficiency often involves integrating Smart Home Devices , such as smart thermostats and lighting, which allow for automated and personalized control. Ultimately, the success of an Energy Management System hinges on the intelligent use of technology to manage energy usage effectively.
Potential Future Developments and Innovations in EMS Technology
Future developments in EMS are likely to focus on enhancing the integration of renewable energy sources, improving grid stability, and increasing the overall resilience of energy systems. We can expect to see more sophisticated energy storage solutions integrated into EMS, enabling better management of intermittent renewable energy sources like solar and wind power. Advanced grid management systems, incorporating AI and ML, will play a crucial role in balancing supply and demand, minimizing power outages, and ensuring grid stability in the face of increasing electrification and decentralized energy generation.
Furthermore, the development of more robust cybersecurity measures will be essential to protect the integrity and reliability of increasingly interconnected energy systems. For instance, blockchain technology could be implemented to enhance the security and transparency of energy transactions within a microgrid.
Effective Energy Management Systems are crucial for modern buildings, impacting both environmental responsibility and operational costs. The integration of such systems often complements the aesthetic choices made in a project’s design, such as those found in Luxury Interior Design , where energy-efficient lighting and climate control are increasingly prioritized to maintain a comfortable and sustainable environment. Ultimately, smart energy management contributes significantly to the overall luxury experience.
Future Applications of EMS in Smart Cities
The application of advanced EMS in smart cities offers significant opportunities for improved sustainability and efficiency.
- Optimized Traffic Management: EMS can analyze traffic patterns and adjust traffic signals to minimize congestion and reduce fuel consumption by vehicles.
- Smart Lighting Systems: AI-powered street lighting systems can automatically adjust brightness based on real-time needs, reducing energy waste and improving public safety.
- Waste Management Optimization: EMS can monitor waste collection routes and optimize schedules, reducing fuel consumption and improving efficiency.
- Renewable Energy Integration: EMS can integrate renewable energy sources like solar and wind power into the city’s energy grid, reducing reliance on fossil fuels.
- Building Energy Management: Centralized EMS can manage energy consumption across multiple buildings, optimizing energy use and reducing carbon emissions.
Case Studies of Energy Management Systems
This section presents a real-world example showcasing the successful implementation of an Energy Management System (EMS), highlighting the achieved energy savings, challenges overcome, and the system’s architecture. The case study demonstrates the transformative impact of EMS on operational efficiency.
Case Study: Energy Optimization at a Large Manufacturing Facility, Energy Management Systems
This case study focuses on a large manufacturing plant that implemented a comprehensive EMS to reduce its energy consumption and improve operational efficiency. Prior to EMS implementation, the facility experienced significant energy waste due to inefficient equipment operation and a lack of real-time monitoring capabilities. The plant’s energy consumption was inconsistent and lacked detailed analysis for effective optimization.
EMS Architecture
The implemented EMS utilized a distributed architecture, incorporating various hardware and software components. The hardware included numerous smart sensors strategically placed throughout the facility to monitor energy usage in real-time. These sensors collected data on electricity consumption, water usage, and temperature. This data was then transmitted wirelessly to a central data acquisition system (DAS). The DAS, a robust server with significant processing power, aggregated and analyzed the data using advanced algorithms.
The software component consisted of a sophisticated supervisory control and data acquisition (SCADA) system, a user-friendly interface for monitoring and control, and advanced analytics software for identifying energy-saving opportunities. The SCADA system allowed for remote monitoring and control of various equipment, enabling optimized operation based on real-time data analysis.
Energy Consumption Patterns: Before and After EMS Implementation
Before EMS implementation, the facility’s energy consumption showed significant peaks and valleys, reflecting inconsistent operational practices. Energy usage was generally high during peak production hours and relatively low during off-peak hours, with no clear correlation between production output and energy usage. After EMS implementation, the energy consumption patterns became significantly more stable and efficient. The EMS optimized equipment operation based on real-time demand and identified opportunities for energy savings, leading to a more consistent and predictable energy consumption profile.
The peaks and valleys were significantly reduced, reflecting a more efficient use of energy resources.
Impact on Operational Efficiency
The EMS implementation resulted in a 15% reduction in overall energy consumption within the first year. This translated into significant cost savings for the facility. Furthermore, the real-time monitoring and control capabilities of the EMS enabled proactive maintenance, reducing downtime and improving overall equipment lifespan. The optimized scheduling of equipment operation based on real-time data improved production efficiency, and the data-driven insights provided by the EMS allowed the facility to make informed decisions about energy-saving upgrades and investments.
The improved operational efficiency also resulted in a reduction in greenhouse gas emissions, aligning with the facility’s sustainability goals. The EMS proved instrumental in transforming the facility’s energy management strategy from a reactive to a proactive approach.
Visual Representation of EMS Data
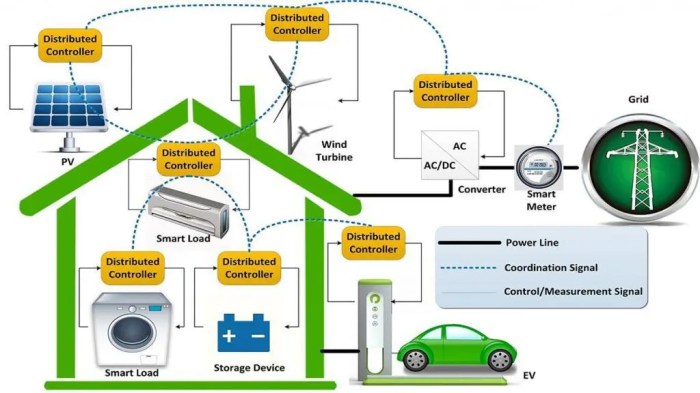
Source: net.au
Effective visualization is crucial for understanding complex energy consumption patterns within a building. A well-designed visual representation allows building managers and energy professionals to quickly identify trends, anomalies, and areas for improvement in energy efficiency. This section details a suitable visual representation and explains its effectiveness in communicating key insights.
A combined stacked area chart and line graph provides a comprehensive overview of energy consumption in a building. The stacked area chart displays the breakdown of energy consumption by source (e.g., heating, cooling, lighting, equipment) over a specified period (e.g., a year or a month). Simultaneously, a line graph overlaid on the area chart shows the total energy consumption.
This dual representation allows for a detailed analysis of both the individual contributions of different energy sources and the overall energy usage trend.
Stacked Area Chart and Line Graph of Energy Consumption
The stacked area chart uses different colors to represent each energy source. For example, heating could be represented by red, cooling by blue, lighting by yellow, and equipment by green. The vertical axis represents the energy consumption in kilowatt-hours (kWh), and the horizontal axis represents the time period (e.g., days, weeks, months). The area of each color segment represents the energy consumed by that specific source.
The line graph, overlaid on the area chart, depicts the total energy consumption across all sources. A clear legend indicates the color code for each energy source. Data labels are displayed on the line graph to highlight significant peaks or troughs in total energy consumption.
This visualization effectively communicates key insights by simultaneously displaying both the overall energy usage and the contribution of each energy source. Building managers can easily identify periods of high energy consumption and pinpoint the specific sources contributing to these peaks. For instance, a sharp increase in the red (heating) segment during winter months highlights the need for optimization of the heating system.
Similarly, unusually high energy consumption from the equipment segment might indicate the need for maintenance or replacement of inefficient equipment.
Justification for Visualization Techniques
The selection of a stacked area chart combined with a line graph is justified by its ability to present multifaceted data in a clear and concise manner. The stacked area chart is effective in showing the proportional contribution of different energy sources to the overall consumption. The line graph provides a clear picture of the overall energy consumption trend, allowing for easy identification of patterns and anomalies.
Overlapping the line graph on the stacked area chart provides a powerful synergy, allowing for a holistic understanding of energy usage. Other visualization methods, such as bar charts or pie charts, might be less effective in showing the temporal dynamics of energy consumption over a period. This combined approach provides a more complete and insightful analysis than using individual charts.
Ultimate Conclusion
In conclusion, Energy Management Systems are not merely technological solutions; they represent a strategic shift towards sustainable and efficient energy practices. By integrating advanced technologies, data analytics, and optimized control strategies, EMS empower organizations and individuals to significantly reduce energy consumption, minimize environmental impact, and maximize return on investment. As technology continues to evolve, the potential of EMS to shape a more sustainable future is undeniable, promising further innovations and wider applications in the years to come.
Clarifying Questions
What is the typical payback period for an EMS investment?
Payback periods vary greatly depending on factors such as the size and type of facility, the specific EMS solution implemented, and energy prices. However, many organizations report payback periods ranging from 1 to 5 years.
Are there any security concerns associated with EMS?
Yes, as with any interconnected system, security is paramount. Robust cybersecurity measures, including access controls, encryption, and regular software updates, are essential to mitigate risks of unauthorized access or data breaches.
How can I choose the right EMS for my needs?
Careful consideration of your facility’s specific energy consumption patterns, budget constraints, and future expansion plans is crucial. Consulting with energy efficiency experts and EMS vendors can help determine the most suitable solution.
What is the role of human intervention in an EMS?
While EMS automate many processes, human oversight remains crucial. Personnel are needed for system monitoring, maintenance, adjustments to control strategies, and troubleshooting.