Carbon Capture and Storage A Comprehensive Overview
Carbon capture and storage (CCS) represents a crucial technology in the global effort to mitigate climate change. This process involves capturing carbon dioxide (CO2) emissions from various sources, transporting them, and storing them securely underground, preventing their release into the atmosphere. Understanding the intricacies of CCS—from its diverse methodologies and applications to its economic and environmental implications—is vital for informed decision-making in the pursuit of a sustainable future.
This exploration delves into the technical aspects, economic considerations, and societal perceptions surrounding this increasingly important technology.
The effectiveness of CCS hinges on a complex interplay of factors. Different capture methods, such as pre-combustion, post-combustion, and oxy-fuel combustion, each present unique advantages and disadvantages regarding efficiency, cost, and energy requirements. Subsequently, the captured CO2 must be transported, often via pipelines or ships, to suitable storage locations. These locations, typically depleted oil and gas reservoirs, saline aquifers, or unmineable coal seams, must be rigorously assessed for their geological suitability and long-term stability to ensure safe and secure storage.
The economic viability of CCS projects is significantly influenced by factors like carbon pricing mechanisms, government policies, and technological advancements. Public perception and social acceptance are equally crucial for successful large-scale deployment.
Methods of Carbon Capture and Storage
Carbon capture and storage (CCS) encompasses a range of technologies designed to prevent carbon dioxide (CO2) emissions from reaching the atmosphere. These methods involve capturing CO2 from various sources, transporting it, and storing it securely for extended periods. The efficacy and environmental impact of each method vary significantly, making careful consideration of all factors crucial for successful implementation.
Carbon Capture Methods
Carbon capture techniques can be broadly categorized as physical or chemical processes. Physical methods rely on changes in pressure and temperature to separate CO2 from other gases. Chemical methods, conversely, utilize chemical reactions to capture CO2, often forming a compound that can be later processed to release the CO2 for storage or utilized in other industrial processes. Examples of physical methods include membrane separation and cryogenic distillation, while chemical methods often involve absorption using amines or adsorption using solid sorbents.
The choice of method depends on factors such as the CO2 concentration in the source gas stream, the operating temperature and pressure, and the overall cost-effectiveness.
Carbon Dioxide Transportation
Once captured, CO2 needs to be transported to a suitable storage location. This typically involves pipelines, but also includes ships and trucks for shorter distances or where pipelines are unavailable. Pipelines offer the most efficient and cost-effective method for large-scale transportation, particularly over long distances. However, the construction and maintenance of extensive pipeline networks represent a significant upfront investment.
Carbon capture and storage (CCS) plays a crucial role in mitigating climate change by trapping CO2 emissions from power plants. However, the production of hydrogen, often reliant on fossil fuels, presents a challenge. A promising solution involves pairing CCS with green hydrogen production methods, as discussed in The future of hydrogen fuel as a green energy source , ensuring a cleaner energy future.
Ultimately, effective CCS implementation is vital for the widespread adoption of hydrogen as a truly sustainable fuel source.
For shorter distances or specific logistical needs, specialized tankers or trucks can transport CO2 in pressurized liquid form. The choice of transportation method depends heavily on geographical factors, the volume of CO2 being transported, and the distance between capture and storage sites.
Geological Storage Options
Several geological formations are suitable for long-term CO2 storage. These include saline aquifers, depleted oil and gas reservoirs, and unmineable coal seams. Saline aquifers, underground formations containing saltwater, are abundant and offer vast storage capacity. Depleted oil and gas reservoirs, having already held hydrocarbons, offer existing infrastructure and geological knowledge, potentially reducing storage costs and risks. Unmineable coal seams can also provide storage, with the added benefit of potentially enhancing coalbed methane recovery.
The suitability of each option depends on geological factors such as porosity, permeability, and depth, as well as proximity to CO2 sources and potential risks associated with leakage.
Comparison of CCS Methods
Method | Efficiency (%) | Cost ($/tonne CO2) | Environmental Impact |
---|---|---|---|
Post-combustion Capture (Amine Absorption) | 85-95 | 50-150 | Moderate energy consumption, potential for amine degradation |
Pre-combustion Capture (Gasification) | 90-95 | 70-180 | Higher capital costs, potential for air pollution during gasification |
Oxy-fuel Combustion | 90-95 | 60-170 | High energy consumption, requires pure oxygen production |
Saline Aquifer Storage | 99+ (assuming no leakage) | 10-50 | Potential for induced seismicity, long-term monitoring required |
Depleted Oil and Gas Reservoirs | 99+ (assuming no leakage) | 20-80 | Potential for leakage, existing infrastructure may reduce costs |
Unmineable Coal Seams | 99+ (assuming no leakage) | 30-100 | Potential for methane release, enhanced coalbed methane recovery possible |
Note: Cost and efficiency figures are estimates and can vary significantly based on specific project parameters and technological advancements. Environmental impacts are summarized and require more detailed assessment on a case-by-case basis.
Applications of Carbon Capture and Storage
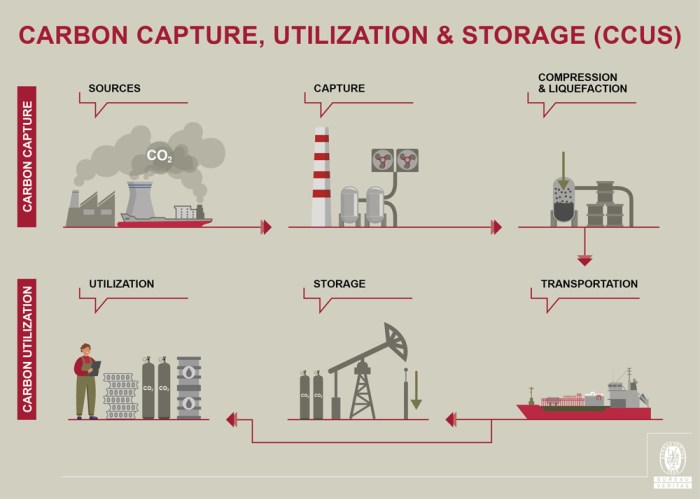
Source: bureauveritas.com
Carbon capture and storage (CCS) plays a crucial role in mitigating climate change by trapping CO2 emissions from power plants and industrial facilities. This technology is a key component within the broader strategy of Green energy technologies for combating climate change , helping to achieve significant reductions in greenhouse gas emissions. Ultimately, the effectiveness of CCS hinges on its integration with other renewable energy solutions and sustainable practices.
Carbon Capture and Storage (CCS) technology offers a crucial pathway towards mitigating climate change and achieving net-zero emissions targets. Its applications span various industrial sectors, playing a vital role in reducing greenhouse gas emissions from both existing and new infrastructure. The successful implementation of CCS hinges on its integration into existing industrial processes and the development of new, efficient technologies.
CCS in Industrial Sectors
CCS is currently being implemented or shows significant potential across numerous industrial sectors. The cement industry, for instance, is a significant emitter of CO2 due to the calcination process. CCS technologies can capture a substantial portion of these emissions before they enter the atmosphere. Similarly, the steel industry, with its high energy consumption and CO2-intensive processes, is exploring CCS as a means of decarbonization.
Other sectors, such as ammonia production and the refining of petroleum products, are also actively investigating and implementing CCS solutions. The successful integration of CCS in these sectors will depend on factors like cost-effectiveness, technological maturity, and regulatory support.
CCS and Climate Change Mitigation
The Intergovernmental Panel on Climate Change (IPCC) highlights CCS as a necessary technology for limiting global warming to well below 2°C, as Artikeld in the Paris Agreement. CCS plays a crucial role in reducing emissions from hard-to-abate sectors where alternative technologies are currently unavailable or impractical at scale. By capturing and storing CO2 emissions, CCS helps to significantly reduce the amount of greenhouse gases entering the atmosphere, thereby mitigating the effects of climate change.
This approach is particularly important for achieving net-zero emissions targets by 2050, a goal set by many countries and organizations worldwide.
CCS in the Energy Sector
The energy sector, including power generation and industrial processes, represents a major source of greenhouse gas emissions. CCS technologies are particularly relevant for power plants fueled by fossil fuels, where capturing CO2 emissions from the flue gas stream can significantly reduce their carbon footprint. In industrial processes, such as those used in the production of hydrogen or chemicals, CCS can capture CO2 emitted during various stages of production.
The deployment of CCS in the energy sector is critical for decarbonizing these sectors and meeting global climate goals. Further research and development are focused on integrating CCS with renewable energy sources, creating a more sustainable energy system.
Successful CCS Project Case Studies
The Sleipner project in Norway, initiated in 1996, is a pioneering example of successful CO2 storage. This project, associated with natural gas production, has demonstrated the safe and reliable storage of millions of tons of CO2 in saline aquifers. Another notable example is the Boundary Dam Power Station in Saskatchewan, Canada, which incorporates CCS technology into a coal-fired power plant.
This project has successfully demonstrated the feasibility of capturing CO2 from a large-scale power generation facility and storing it underground. These projects, while not without their challenges, offer valuable lessons and insights for future CCS deployments. They underscore the technological viability and the potential environmental benefits of CCS technology.
Technological Challenges and Advancements in Carbon Capture and Storage
Large-scale deployment of carbon capture and storage (CCS) technologies faces significant hurdles, demanding continuous innovation and improvement to achieve widespread adoption and meaningful climate impact. These challenges span technological, economic, and societal aspects, requiring a multifaceted approach to overcome them. This section delves into the key technological obstacles and the ongoing advancements designed to enhance efficiency and affordability.
Key Technological Challenges in CCS Deployment
Several critical technological challenges hinder the widespread implementation of CCS. High capital and operational costs are a major barrier, particularly for smaller-scale applications. Energy penalties associated with capturing CO2 can significantly reduce the overall efficiency of power plants and industrial processes. The transportation and storage of captured CO2 present logistical complexities and safety concerns, requiring robust infrastructure and monitoring systems.
Furthermore, the long-term stability and security of geological storage sites need to be rigorously assessed and ensured to prevent CO2 leakage. Finally, the development of suitable capture technologies for diverse emission sources, including those with low CO2 concentrations, remains a challenge.
Advancements in Carbon Capture Technology Efficiency and Cost Reduction
Research and development efforts are actively focused on improving the efficiency and reducing the cost of CCS technologies. Significant progress is being made in developing more energy-efficient capture solvents and membranes. The use of advanced materials and process optimization techniques are continuously explored to minimize energy consumption and capital expenditures. Innovations in CO2 compression and transportation, including the use of pipelines and ships optimized for CO2 transport, are aimed at reducing logistical costs.
Moreover, the exploration of novel storage sites and improved monitoring techniques are crucial to enhance the safety and long-term reliability of geological storage. For example, the development of hybrid capture technologies that combine different methods to exploit the advantages of each and overcome individual limitations is a promising area of research.
Comparison of Carbon Capture Technologies
Several carbon capture technologies exist, each with its own advantages and disadvantages. Post-combustion capture, which involves capturing CO2 from flue gases after combustion, is a mature technology applicable to existing power plants and industrial facilities, but it has a relatively high energy penalty. Pre-combustion capture, where CO2 is separated before combustion, offers higher capture efficiency but requires modifications to existing infrastructure.
Oxy-fuel combustion, which involves burning fuel in pure oxygen, produces a CO2-rich stream that is easier to capture, but it requires significant modifications to existing power plants. Membrane separation, a promising technology, utilizes membranes to selectively separate CO2 from other gases, but its scalability and cost-effectiveness are still under development. Each technology’s suitability depends on factors such as the specific application, emission source characteristics, and economic considerations.
For instance, post-combustion capture is often favoured for retrofitting existing facilities due to its adaptability, while pre-combustion capture is more suitable for new installations.
Hypothetical CCS System for a Cement Plant
Consider a cement plant employing a post-combustion capture system. The system would consist of: (1) A flue gas cleaning unit to remove particulate matter and other pollutants from the exhaust gases. (2) A CO2 capture unit employing an amine-based solvent to absorb CO2 from the cleaned flue gas. (3) A CO2 regeneration unit to release the captured CO2 from the solvent, preparing it for transportation and storage.
(4) A compression unit to compress the purified CO2 to a suitable pressure for pipeline transport. (5) A pipeline network to transport the compressed CO2 to a suitable geological storage site. (6) A geological storage site with monitoring systems to ensure long-term storage security. Operational procedures would involve continuous monitoring of all system components, regular maintenance, and periodic safety inspections.
The captured CO2 would be injected into the storage site under careful supervision, with continuous monitoring for leakage or other anomalies. The specific design and operational parameters would depend on the plant’s size, emission rate, and the characteristics of the chosen storage site. Such a system would aim to minimize energy penalties through process optimization and efficient solvent regeneration, and prioritize safety and environmental protection throughout the entire process.
Economic and Policy Aspects of Carbon Capture and Storage
The economic viability and widespread adoption of carbon capture and storage (CCS) technologies are intricately linked to a complex interplay of factors, including initial investment costs, ongoing operational expenses, and the effectiveness of government policies designed to incentivize their use. A comprehensive understanding of these economic and policy aspects is crucial for the successful deployment of CCS in mitigating climate change.
Economic Viability of CCS Projects
The economic feasibility of CCS projects is highly dependent on a variety of factors. Capital costs, encompassing the expenses associated with building and installing the necessary infrastructure, can be substantial, varying significantly depending on the specific technology employed and the scale of the project. Operating expenses, which include energy consumption, maintenance, and personnel costs, represent a continuous financial burden.
Furthermore, the cost-effectiveness of CCS is heavily influenced by the price of carbon, either through direct carbon taxes or emissions trading schemes (ETS). A higher carbon price makes CCS more economically attractive, as the revenue generated from avoided carbon emissions offsets some of the project’s costs. For example, a large-scale CCS project integrated into a power plant might require billions of dollars in upfront investment, with annual operating costs potentially reaching tens of millions.
Carbon capture and storage (CCS) technologies are crucial for mitigating climate change, particularly from fossil fuel sources. However, the transition to a cleaner energy future relies heavily on expanding renewable energy sources, as detailed in this informative overview of Renewable energy projects in the US. Ultimately, a comprehensive approach combining CCS with a robust renewable energy infrastructure will be essential to achieving significant emissions reductions.
However, if a robust carbon pricing mechanism is in place, the revenue generated from carbon credits could significantly improve the project’s return on investment. Conversely, in the absence of a strong carbon price, the project’s economic viability would be severely challenged.
Government Policies and Regulations Promoting CCS Adoption
Government intervention plays a pivotal role in shaping the landscape for CCS deployment. Policies and regulations can significantly influence the economic attractiveness of CCS projects. These interventions can take various forms, including direct financial subsidies, tax breaks, grants, and loan guarantees to reduce the financial risk associated with large-scale CCS projects. Regulatory frameworks mandating carbon emissions reductions can also create a market demand for CCS technologies, thereby driving investment and innovation.
For instance, many countries have implemented carbon taxes or participate in ETS programs, creating a financial incentive for industries to reduce their carbon footprint, making CCS a more attractive option. Moreover, governments can streamline permitting processes and offer regulatory certainty, reducing the bureaucratic hurdles and uncertainties that often deter private investment in CCS. The establishment of clear and consistent policy frameworks is essential to foster long-term investment and confidence in the CCS sector.
Economic Incentives and Barriers to Widespread CCS Deployment
Several economic incentives and barriers influence the widespread adoption of CCS technologies. Incentives include the aforementioned government subsidies, carbon pricing mechanisms, and potential revenue streams from selling carbon credits. However, substantial barriers remain. The high capital costs and operational expenses associated with CCS are major deterrents. Technological challenges, such as the energy intensity of some CCS methods and the need for safe and secure geological storage, also contribute to the high costs.
Furthermore, the lack of a well-established market for carbon credits in some regions and the uncertainty surrounding future carbon pricing policies can hinder investment decisions. The risk associated with geological storage, including potential leakage of captured CO2, also presents a significant barrier, demanding robust monitoring and verification mechanisms. The integration of CCS into existing industrial processes can also be complex and expensive, further increasing the barriers to adoption.
Influence of Carbon Pricing Mechanisms on CCS Economic Feasibility, Carbon capture and storage
Carbon pricing mechanisms are instrumental in influencing the economic feasibility of CCS. Mechanisms like carbon taxes and emissions trading schemes directly affect the cost-benefit analysis of CCS projects. Higher carbon prices increase the value of avoided emissions, making CCS more economically attractive. Conversely, low or absent carbon prices reduce the economic viability of CCS, rendering it less competitive compared to alternative emission reduction strategies.
For example, if a carbon tax is set at $50 per ton of CO2, a power plant capturing and storing 1 million tons of CO2 annually would generate $50 million in revenue from carbon credits, potentially offsetting a significant portion of its operating costs. The design and implementation of effective carbon pricing mechanisms are crucial for ensuring the economic competitiveness of CCS and its contribution to climate change mitigation efforts.
Carbon capture and storage (CCS) is crucial for mitigating climate change, but its effectiveness hinges on reliable energy storage for intermittent renewable sources. Further advancements are needed to make CCS truly impactful, particularly in integrating it with innovative solutions discussed in this article on Innovations in green energy storage systems. Ultimately, the success of CCS depends heavily on the parallel development of efficient and scalable green energy storage technologies.
The level of the carbon price needs to be sufficient to overcome the high capital and operational costs associated with CCS deployment.
Environmental Considerations of Carbon Capture and Storage
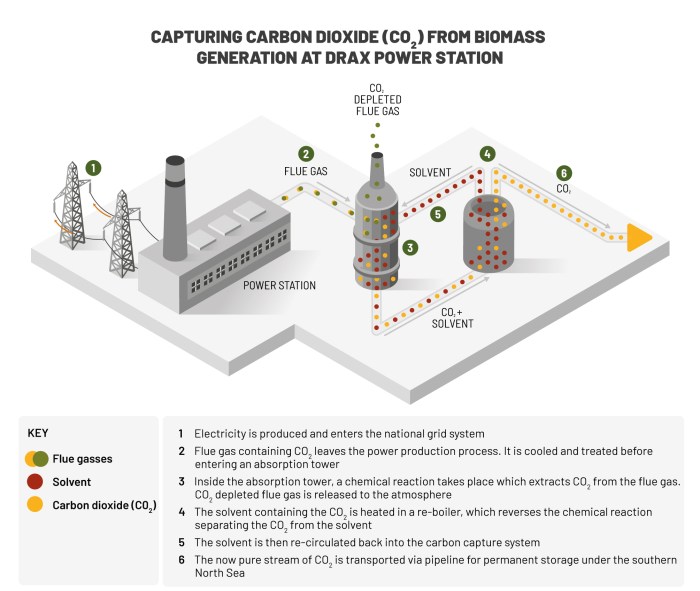
Source: ctfassets.net
Carbon capture and storage (CCS) presents a multifaceted approach to mitigating climate change, but its implementation necessitates a thorough understanding of its potential environmental impacts. While CCS aims to reduce greenhouse gas emissions, the process itself and the long-term storage of CO2 introduce a range of environmental considerations that must be carefully evaluated. These considerations extend beyond the immediate effects of CO2 capture and encompass the entire lifecycle of the technology, including site selection, monitoring, and potential risks.
CO2 Leakage and Storage Site Selection Risks
The primary environmental concern surrounding CCS is the potential for CO2 leakage from storage sites. This leakage could occur through various pathways, including faults, fractures in the geological formations, or even through wellbore integrity issues. The consequences of leakage could be significant, contributing to atmospheric greenhouse gas concentrations and potentially impacting local ecosystems through changes in soil and groundwater chemistry.
Careful site selection is therefore crucial, involving extensive geological surveys to identify suitable storage formations with low permeability and minimal risk of leakage. Factors such as the depth and capacity of the formation, the presence of overlying impermeable caprocks, and the proximity to sensitive ecosystems are all carefully assessed. For instance, depleted oil and gas reservoirs, saline aquifers, and unmineable coal seams are often considered, with detailed modeling used to predict the long-term behavior of CO2 within the chosen site.
Failure to adequately assess these risks could lead to environmental damage and undermine the effectiveness of CCS.
Life-Cycle Assessment of CCS Technologies
The environmental impact of CCS extends beyond the storage phase and encompasses the entire lifecycle of the technology. A life-cycle assessment (LCA) considers energy consumption and greenhouse gas emissions associated with each stage, from CO2 capture at the emission source to transportation and storage. Different CCS technologies exhibit varying environmental footprints. For example, post-combustion capture, which involves separating CO2 from flue gases after combustion, typically requires more energy than pre-combustion capture, where CO2 is separated before combustion.
This energy consumption translates to additional greenhouse gas emissions, potentially offsetting some of the climate benefits of CO2 storage. Similarly, the transportation of captured CO2 to storage sites can generate significant emissions, depending on the distance and mode of transport. Therefore, a comprehensive LCA is essential to evaluate the net environmental benefit of different CCS technologies and to identify areas for improvement in efficiency and sustainability.
The aim is to minimize the overall environmental footprint, ensuring that the climate benefits outweigh the environmental costs associated with the CCS process.
Monitoring and Verification of CO2 Storage Sites
Ensuring the long-term security of CO2 storage sites is paramount. This requires robust monitoring and verification strategies to detect any potential leakage and assess the integrity of the storage formation. Monitoring techniques include geophysical methods, such as seismic surveys and gravity measurements, to detect changes in subsurface pressure and CO2 saturation. Geochemical monitoring of groundwater and soil samples can also be used to detect the presence of leaked CO2.
Furthermore, regular inspections of wellbores and other infrastructure are crucial to identify and address any potential integrity issues. The data collected through these monitoring efforts are used to verify the long-term security of the storage site and to inform any necessary remediation measures. This continuous monitoring and verification process is vital to build public confidence in CCS and to ensure its long-term effectiveness in mitigating climate change.
Real-time monitoring systems coupled with advanced data analytics are crucial in providing early warning systems and facilitating timely responses to any potential leakage incidents.
Potential Environmental Benefits Compared to Other Mitigation Strategies
While CCS presents environmental challenges, it also offers potential benefits compared to other greenhouse gas mitigation strategies. In certain industrial sectors, such as cement and steel production, where CO2 emissions are difficult to eliminate entirely through other means, CCS can play a significant role in reducing emissions. Furthermore, CCS can be integrated with other mitigation strategies, such as renewable energy and energy efficiency improvements, to create a more comprehensive approach to climate change mitigation.
Compared to simply relying on afforestation or other carbon sequestration methods, CCS offers a more direct and potentially faster pathway to reducing atmospheric CO2 concentrations, particularly in sectors with significant point-source emissions. The effectiveness of CCS as a climate mitigation strategy depends heavily on its responsible and sustainable implementation, emphasizing rigorous environmental assessment and monitoring throughout its lifecycle.
Public Perception and Social Acceptance of Carbon Capture and Storage
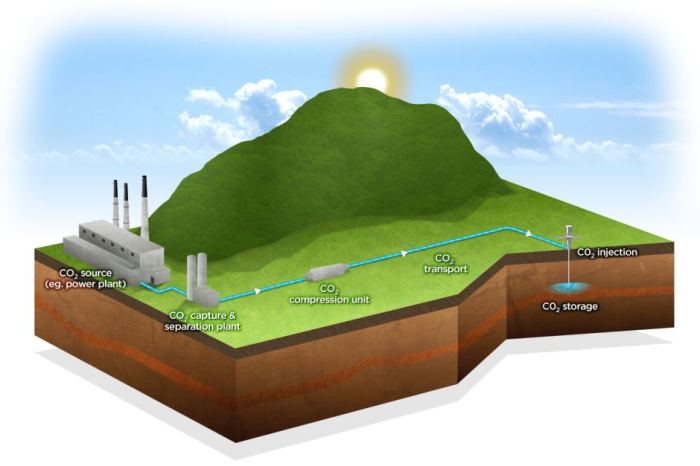
Source: ac.id
Public perception of carbon capture and storage (CCS) is complex and multifaceted, influenced by a range of factors including media portrayals, personal values, and trust in technology and governing bodies. While CCS offers a potentially crucial tool in mitigating climate change, widespread public acceptance is essential for its successful deployment. Addressing public concerns and fostering trust is therefore paramount.Public perceptions of CCS are often shaped by a lack of understanding of the technology and its potential benefits.
Concerns frequently center on the safety of CO2 storage, potential environmental impacts, and the economic viability of CCS projects. These concerns, often amplified by misinformation or negative media coverage, can lead to public opposition and hinder project development. Furthermore, a perceived lack of transparency and community engagement in project planning can exacerbate these issues.
Public Concerns Regarding CCS Technologies
Several key concerns consistently emerge in public discourse surrounding CCS. Safety concerns regarding CO2 leakage from storage sites are prominent, fueled by fears of potential groundwater contamination or atmospheric release. Environmental impacts, such as habitat disruption during construction or operation, also feature heavily. Economic anxieties often revolve around the perceived high costs of CCS implementation and the potential for financial burdens on taxpayers or communities hosting CCS facilities.
Finally, a lack of trust in government regulations and the companies involved in CCS projects further contributes to public apprehension. These concerns are not necessarily unfounded; careful risk assessment, transparent communication, and robust regulatory frameworks are crucial to address them effectively.
Strategies for Improving Public Understanding and Acceptance of CCS
Effective communication is vital for building public trust and acceptance of CCS. This involves employing a multi-pronged approach that utilizes various channels and techniques to reach diverse audiences. Clear, accessible information about the science and technology behind CCS, presented in a non-technical manner, is essential. Community engagement initiatives, such as public forums and workshops, provide opportunities for direct dialogue between project developers and the public, allowing for concerns to be addressed and misconceptions to be clarified.
Transparency in project planning and decision-making is also crucial, ensuring that communities feel involved and informed throughout the process. Furthermore, highlighting successful CCS projects and their positive impacts can help to counter negative narratives and demonstrate the technology’s viability. For example, the Sleipner project in Norway, a pioneering CCS venture, has successfully demonstrated the long-term storage of CO2 in saline aquifers, providing a real-world example of CCS’s potential.
Potential Social and Community Impacts of CCS Projects
CCS projects can have significant social and community impacts, both positive and negative. Potential benefits include job creation in construction, operation, and maintenance, as well as economic stimulus through investment and increased local tax revenue. However, negative impacts may also arise, including displacement of communities or disruption of local ecosystems. Careful consideration of these potential impacts is necessary during project planning, involving thorough environmental impact assessments and community consultation to mitigate potential negative consequences and maximize positive benefits.
A transparent process that ensures fair compensation and equitable distribution of benefits is crucial for fostering community acceptance. For instance, the development of a CCS facility could lead to an influx of skilled workers, boosting local employment and economic activity. Conversely, concerns about potential environmental damage or safety risks could lead to community resistance, even if the project offers economic benefits.
A Plan for Effective Communication and Engagement with Stakeholders Regarding CCS
An effective communication and engagement plan requires a multi-stage approach. Initially, a thorough stakeholder analysis should identify all relevant groups, including local communities, environmental organizations, industry representatives, and government agencies. Tailored communication strategies should then be developed to address the specific concerns and needs of each stakeholder group. This includes utilizing diverse communication channels, such as websites, social media, community meetings, and educational materials.
Regular feedback mechanisms should be established to ensure that communication is two-way and responsive to stakeholder input. Transparency in decision-making and project implementation is crucial for building trust. Finally, independent third-party verification of safety and environmental performance can help to alleviate concerns and build confidence in the technology. A well-structured plan, emphasizing transparency, engagement, and proactive communication, is crucial to fostering public acceptance of CCS.
Closing Notes
In conclusion, carbon capture and storage presents a multifaceted approach to addressing climate change. While technological challenges and economic considerations remain, ongoing research and development, coupled with supportive policies, are paving the way for wider adoption. Successful implementation requires a comprehensive understanding of the technological intricacies, economic implications, environmental considerations, and societal perspectives. Ultimately, the future of CCS hinges on a collaborative effort involving governments, industries, researchers, and the public to ensure its safe, effective, and sustainable deployment as a vital tool in the fight against climate change.
The potential benefits for a sustainable future are substantial, demanding a continued commitment to innovation and responsible implementation.
User Queries: Carbon Capture And Storage
What are the main risks associated with CO2 leakage from storage sites?
Potential risks include localized environmental damage, such as soil and water contamination, and the release of CO2 into the atmosphere, negating the climate benefits of CCS. Rigorous site selection, monitoring, and well integrity management are crucial to mitigate these risks.
How does CCS compare to other greenhouse gas mitigation strategies, such as renewable energy?
CCS is often considered a complementary technology to renewable energy sources. While renewables aim to reduce emissions at the source, CCS addresses emissions from existing and hard-to-abate industrial processes. A balanced approach combining both is crucial for effective climate change mitigation.
What is the current state of CCS deployment globally?
While CCS deployment is still relatively limited, several large-scale projects are operational worldwide, demonstrating the technology’s feasibility. However, significant expansion is needed to achieve meaningful climate impact.
What role does carbon pricing play in the economic feasibility of CCS?
Carbon pricing mechanisms, such as carbon taxes or emissions trading schemes, can significantly improve the economic viability of CCS by creating a market value for avoided emissions. Higher carbon prices make CCS projects more competitive.