The Future of Hydrogen Fuel as a Green Energy Source
The future of hydrogen fuel as a green energy source is rapidly evolving, presenting both immense opportunities and significant challenges. This exploration delves into the multifaceted aspects of hydrogen energy, from its production methods and storage solutions to its diverse applications and the crucial economic and policy considerations shaping its future. We will examine the technological advancements driving progress, the environmental impacts, and the potential for hydrogen to play a pivotal role in achieving global decarbonization goals.
The journey towards a hydrogen-powered future requires a comprehensive understanding of the entire value chain, from the sustainable generation of hydrogen to its efficient utilization in fuel cells across various sectors. This examination will analyze the various hurdles that must be overcome, including the development of cost-effective and scalable production techniques, safe and efficient storage and transportation methods, and the widespread adoption of fuel cell technology.
Furthermore, we’ll investigate the economic viability and the essential role of supportive government policies in fostering the growth of this promising energy source.
Hydrogen Production Methods
Producing hydrogen, a versatile energy carrier, involves several methods, each with its own advantages, disadvantages, and environmental footprint. The choice of method significantly impacts the overall sustainability and economic viability of hydrogen as a fuel source. A key distinction lies between “grey” hydrogen, produced from fossil fuels, and “green” hydrogen, generated using renewable energy sources.
Different methods exist for producing hydrogen, each characterized by its energy source, efficiency, and environmental impact. Understanding these nuances is crucial for the responsible development and deployment of hydrogen technologies.
Hydrogen Production Method Comparison
The following table summarizes the key characteristics of three common hydrogen production methods: electrolysis, steam methane reforming (SMR), and gasification.
Method | Energy Source | Environmental Impact | Efficiency |
---|---|---|---|
Electrolysis | Electricity (renewable or non-renewable) | Low (with renewable electricity); High (with fossil fuel-based electricity)primarily related to greenhouse gas emissions from electricity generation. Water consumption is also a factor. | Generally high, improving with technological advancements. Efficiency depends on the electrolyzer type and operating conditions. |
Steam Methane Reforming (SMR) | Natural gas (methane) | High – significant greenhouse gas emissions (CO2, CH4) and air pollutants. | Relatively high, but lower than electrolysis when using renewable electricity. |
Gasification | Coal, biomass, or waste | Variable – depends heavily on the feedstock. Coal gasification has high emissions; biomass gasification can be carbon-neutral or even carbon-negative if sustainably sourced. | Moderate; can be improved through process optimization and carbon capture technologies. |
Advancements and Challenges in Green Hydrogen Production
The development of cost-effective and sustainable green hydrogen production relies on significant advancements in several key areas. These advancements are critical to making green hydrogen a competitive and environmentally responsible energy solution.
Significant progress is being made in improving the efficiency and reducing the cost of electrolyzers, particularly alkaline and proton exchange membrane (PEM) electrolyzers. Research into novel materials and designs aims to enhance their performance and durability. However, challenges remain in scaling up production, ensuring reliable operation, and reducing the overall cost of green hydrogen. The intermittent nature of renewable energy sources also poses a challenge, requiring efficient energy storage solutions or strategies to manage supply and demand.
Hypothetical Large-Scale Green Hydrogen Production Facility
A large-scale green hydrogen production facility would require a robust infrastructure, incorporating renewable energy sources, efficient electrolyzers, and effective waste management strategies. This hypothetical facility illustrates the key components and considerations for such a project.
Imagine a facility located in a region with abundant solar and wind resources, such as parts of the southwestern United States or certain areas of Australia. The facility would utilize a combination of photovoltaic (PV) solar panels and wind turbines to generate electricity. This electricity would power a large array of PEM electrolyzers, chosen for their high efficiency and relatively fast response times.
A significant portion of the facility would be dedicated to the electrolyzer stacks, along with associated control systems and power electronics. A sophisticated water management system would ensure a continuous supply of purified water to the electrolyzers and handle wastewater treatment. The produced green hydrogen would be compressed and stored in high-pressure tanks or potentially liquefied for easier transportation.
A comprehensive waste management plan would address any byproducts from the water treatment process and ensure minimal environmental impact. The facility would be designed to integrate seamlessly with existing or planned transmission infrastructure to distribute the hydrogen to end-users.
Hydrogen Storage and Transportation
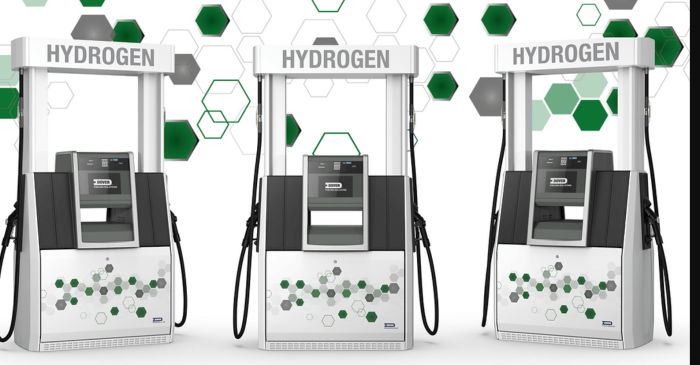
Source: hydrogen-central.com
Efficient and safe storage and transportation are crucial for the widespread adoption of hydrogen as a clean energy source. The challenges lie in hydrogen’s low energy density compared to other fuels, its potential for embrittlement of certain materials, and the associated costs of infrastructure development. Solutions involve developing advanced materials and optimizing existing technologies to enhance safety, reduce costs, and improve efficiency.Hydrogen’s unique properties necessitate specialized storage and transport methods.
The choice of method depends on factors like the quantity of hydrogen, the distance of transport, and the intended application. A balance must be struck between safety, cost-effectiveness, and energy efficiency.
Hydrogen Storage Methods
Several methods exist for storing hydrogen, each with its own advantages and disadvantages. The selection of a suitable method depends on the specific application and its requirements.
- Compressed Gas Storage: Hydrogen is stored at high pressures (typically 200-700 bar) in strong, lightweight cylinders. This method is relatively mature and widely used, but it suffers from lower energy density compared to other methods. The high pressure necessitates robust and expensive storage tanks.
- Liquid Hydrogen Storage: Hydrogen is liquefied at -253°C, significantly reducing its volume. This method offers higher energy density than compressed gas storage, but requires significant energy input for liquefaction and suffers from boil-off losses during storage and transport. Cryogenic tanks are expensive and require specialized insulation.
- Metal Hydrides Storage: Hydrogen is absorbed by certain metals or alloys, forming metal hydrides. This method offers relatively high storage density at ambient temperatures and pressures, but the weight of the metal hydride system can be significant, and the absorption and desorption processes can be slow and energy-intensive. Research is ongoing to find more efficient and cost-effective metal hydride materials.
Hydrogen Transportation Methods
The transportation of hydrogen presents unique challenges due to its low density and flammability. Various methods are employed, each with its own strengths and weaknesses.
- Pipelines: Pipelines are an efficient way to transport large volumes of hydrogen over long distances. However, the construction of dedicated hydrogen pipelines requires significant investment, and hydrogen’s embrittling effect on certain steels necessitates the use of specialized materials, increasing costs.
- Tankers: Specialized cryogenic tankers are used for maritime transport of liquefied hydrogen. This method is suitable for long-distance transport but is expensive and requires significant infrastructure for handling liquid hydrogen. The potential for boil-off losses during transport needs to be considered.
- Trucks: Trucks are commonly used for shorter-distance transport of compressed hydrogen in high-pressure cylinders. This method offers flexibility but is less efficient for large-scale transportation due to higher transportation costs per unit of hydrogen.
Secure Hydrogen Storage and Transportation System for Fueling Stations
Imagine a hydrogen fueling station designed for passenger vehicles. The system begins with a secure, above-ground storage tank containing liquid hydrogen. This tank is double-walled, with vacuum insulation to minimize boil-off. The tank is equipped with pressure relief valves and other safety features to prevent overpressure or leaks. Liquid hydrogen is pumped from the storage tank through a vaporizer, converting it into gaseous hydrogen.
The gaseous hydrogen then passes through a series of filters and regulators to ensure purity and control pressure before dispensing to vehicles. The entire system is housed within a secure enclosure, equipped with leak detection systems and emergency shut-off valves. Hydrogen is transported to the fueling station via a dedicated pipeline, ensuring a constant supply of fuel.
The pipeline is made of materials resistant to hydrogen embrittlement and is regularly inspected for leaks. For safety, the fueling station is located away from residential areas and has emergency response plans in place. The entire system is monitored remotely to ensure optimal operation and safety.
Hydrogen Fuel Cell Technology
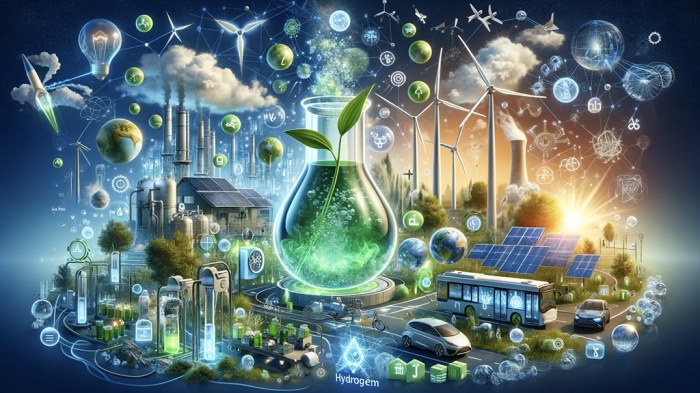
Source: electrum.pl
Hydrogen fuel cells represent a pivotal technology in the transition to a cleaner energy future. They offer a highly efficient and environmentally friendly way to convert the chemical energy stored in hydrogen into electricity, producing only water as a byproduct. This technology is poised to play a significant role in various applications, from powering vehicles to providing stationary power generation.
At its core, a hydrogen fuel cell operates through an electrochemical process, effectively acting as a reversed electrolysis cell. Hydrogen gas is fed into the anode (negative electrode), while oxygen (from the air) is fed into the cathode (positive electrode). Within the fuel cell, a catalyst facilitates the splitting of hydrogen molecules into protons and electrons. The protons pass through an electrolyte membrane to the cathode, while the electrons travel through an external circuit, generating a direct current (DC) electricity.
At the cathode, the protons, electrons, and oxygen combine to form water. This process is highly efficient, converting a significant portion of the hydrogen’s chemical energy directly into electricity, unlike combustion engines which lose much energy as heat.
A hydrogen fuel cell essentially uses a chemical reaction between hydrogen and oxygen to produce electricity and water, cleanly and efficiently.
Hydrogen Fuel Cell Components and Material Science Challenges
A hydrogen fuel cell comprises several key components: the anode, the cathode, the electrolyte membrane, and bipolar plates (in stacks). The electrolyte membrane is crucial, acting as a selective barrier that allows protons to pass through but prevents the passage of electrons. Materials science plays a vital role in enhancing fuel cell performance. Current challenges revolve around improving the durability of the membrane, particularly its resistance to degradation under high temperatures and pressures.
Developing more efficient and cost-effective catalysts, such as platinum-based materials, is also critical. Research focuses on reducing the reliance on expensive platinum by exploring alternative catalyst materials and optimizing catalyst layer structures. Improving the conductivity and corrosion resistance of bipolar plates, which distribute current and reactants, is another important area of focus. For example, significant research efforts are focused on developing more durable and efficient membranes based on perfluorinated sulfonic acid polymers (PFSA) or alternative materials like alkaline membranes, aiming to reduce the reliance on platinum and improve long-term stability.
Types of Hydrogen Fuel Cells and Their Applications, The future of hydrogen fuel as a green energy source
Different types of hydrogen fuel cells exist, each with its own characteristics and applications. Proton exchange membrane (PEM) fuel cells are the most prevalent type, particularly in transportation applications due to their relatively low operating temperature and rapid start-up time. However, PEM fuel cells typically require pure hydrogen and can be sensitive to contaminants. Solid oxide fuel cells (SOFCs), on the other hand, operate at much higher temperatures, allowing for higher efficiency and the use of less expensive catalysts.
Their high operating temperatures, however, require longer start-up times and present material challenges. PEM fuel cells are commonly used in electric vehicles and portable power devices, while SOFCs find applications in stationary power generation and potentially in large-scale power plants due to their higher efficiency at higher temperatures. Other types, such as alkaline fuel cells (AFCs) and direct methanol fuel cells (DMFCs), also exist, each with its own advantages and disadvantages depending on the specific application.
For instance, while AFCs offer high efficiency, they are sensitive to carbon dioxide and require highly purified reactants, limiting their widespread applicability compared to PEM fuel cells.
Hydrogen Fuel Cell Applications
Hydrogen fuel cells, converting chemical energy directly into electricity, offer a promising pathway towards a cleaner energy future. Their versatility allows for application across diverse sectors, presenting significant opportunities for decarbonization and improved air quality. This section will explore current and potential applications, highlighting their advantages and challenges.
Current and Potential Applications of Hydrogen Fuel Cells
The following table summarizes the diverse applications of hydrogen fuel cells across various sectors, outlining their benefits and associated hurdles.
Sector | Application | Advantages | Challenges |
---|---|---|---|
Transportation | Fuel cell electric vehicles (FCEVs), buses, trains, ships | Zero tailpipe emissions, long range, fast refueling, quiet operation | High initial cost, limited refueling infrastructure, hydrogen production costs |
Energy Storage | Grid-scale energy storage, backup power systems, residential energy storage | High energy density, efficient energy conversion, long lifespan, silent operation | Cost competitiveness with other storage technologies, hydrogen storage and transportation infrastructure |
Industrial Processes | Power generation for factories, material processing, heavy machinery | Reduced reliance on fossil fuels, improved air quality, potential for distributed generation | High capital costs, integration challenges with existing infrastructure, safety considerations |
Portable Power | Laptops, mobile phones, remote sensing equipment | High power density, quiet operation, long operational life | Cost, size and weight limitations compared to batteries |
Impact on Greenhouse Gas Emissions and Air Quality
Widespread adoption of hydrogen fuel cell technology could significantly reduce greenhouse gas emissions and improve air quality, particularly in urban areas. Replacing gasoline-powered vehicles with FCEVs would eliminate tailpipe emissions of greenhouse gases and pollutants like nitrogen oxides and particulate matter, leading to cleaner air and a healthier environment. Similarly, the use of hydrogen fuel cells in power generation would decrease reliance on fossil fuel-based electricity production, contributing to a substantial reduction in overall carbon emissions.
For example, studies have shown that replacing diesel buses with hydrogen fuel cell buses in a city like London could lead to a considerable reduction in local air pollution and greenhouse gas emissions, improving public health and environmental quality.
Decarbonization of Specific Industries
Hydrogen fuel cells hold significant potential for decarbonizing heavy-duty trucking and maritime shipping, sectors currently reliant on fossil fuels. In heavy-duty trucking, hydrogen fuel cells offer a viable alternative to diesel engines, providing the necessary power and range for long-haul transportation while significantly reducing greenhouse gas emissions. Similarly, the maritime industry, a major contributor to greenhouse gas emissions, could benefit from the adoption of hydrogen fuel cell technology for powering ships, reducing their environmental footprint.
Companies like Toyota and Hyundai are already investing heavily in developing hydrogen fuel cell powered trucks, and several shipping companies are exploring the feasibility of hydrogen-powered vessels, demonstrating the growing interest and investment in this technology for decarbonizing these crucial sectors. The successful implementation of these technologies requires overcoming challenges related to hydrogen storage and transportation, as well as the development of robust and cost-effective fuel cell systems specifically designed for these demanding applications.
Economic and Policy Aspects: The Future Of Hydrogen Fuel As A Green Energy Source
The economic viability of hydrogen fuel cell technology hinges on a complex interplay of production costs, infrastructure development, and market demand. Currently, green hydrogen production, particularly via electrolysis powered by renewable energy, is significantly more expensive than hydrogen produced from fossil fuels. This cost disparity is a major barrier to widespread adoption. However, technological advancements, economies of scale, and supportive government policies are gradually shifting the economic landscape.The current high cost of green hydrogen production stems from several factors including the cost of renewable energy, the efficiency of electrolyzers, and the cost of hydrogen storage and transportation.
Infrastructure investment, encompassing production facilities, pipelines, refueling stations, and storage solutions, represents a substantial capital expenditure. Market demand, while growing, remains relatively limited compared to established energy sources, leading to higher initial production costs. Addressing these economic challenges is crucial for realizing the full potential of hydrogen as a green energy source.
Current Economic Viability of Hydrogen Fuel Cell Technology
The economic viability of hydrogen fuel cell technology is currently limited by several factors. Production costs for green hydrogen remain significantly higher than for grey hydrogen (produced from natural gas). The lack of widespread infrastructure necessitates substantial investment in pipelines, refueling stations, and storage facilities. Furthermore, the relatively small market size limits economies of scale, making the technology less competitive compared to established energy sources.
However, technological advancements and government support are steadily improving the economics of hydrogen fuel cells. For example, cost reductions in renewable energy generation, particularly solar and wind power, are directly impacting the cost of green hydrogen production. Similarly, improvements in electrolyzer technology are increasing efficiency and reducing production costs. As market demand grows, economies of scale will further reduce the cost of hydrogen production and infrastructure development.
A realistic assessment needs to consider the long-term cost benefits of reduced carbon emissions and improved energy security, factors that are not always fully reflected in short-term economic analyses.
Government Policies to Accelerate Hydrogen Adoption
Several government policies can significantly accelerate the adoption of hydrogen fuel cell technology. These policies should address the cost competitiveness of hydrogen, stimulate investment in infrastructure, and encourage market demand.
- Subsidies and tax credits for green hydrogen production: Direct financial support can make green hydrogen production more competitive with fossil fuel-based alternatives.
- Investment tax credits for hydrogen refueling infrastructure: Incentivizing the development of a nationwide hydrogen refueling network is essential for widespread adoption.
- Mandates for hydrogen vehicle purchases: Government mandates can create a guaranteed market for hydrogen fuel cell vehicles, encouraging production and infrastructure development.
- Research and development funding for hydrogen technology: Continued investment in research and development is crucial for improving the efficiency and reducing the cost of hydrogen production and fuel cell technology.
- Carbon pricing mechanisms: Implementing a carbon tax or cap-and-trade system can make fossil fuels less competitive and incentivize the adoption of cleaner alternatives like hydrogen.
The Role of Government Regulations and Incentives
Government regulations and incentives play a crucial role in shaping the development and deployment of hydrogen energy infrastructure. Regulations related to safety standards, emissions, and environmental impact are essential for ensuring the responsible and sustainable development of hydrogen technologies. Incentives such as tax credits, grants, and loan guarantees can stimulate private investment in hydrogen production, storage, and transportation infrastructure.
Furthermore, government procurement policies can create a significant early market for hydrogen fuel cell vehicles and other hydrogen-based technologies, fostering innovation and accelerating cost reductions. A coordinated approach involving both regulatory frameworks and financial incentives is necessary to create a favorable environment for the growth of the hydrogen economy.
Hypothetical Policy Framework for Green Hydrogen
A hypothetical policy framework to stimulate investment in green hydrogen production and fuel cell technology could include a tiered system of incentives based on the level of green hydrogen production and the integration of renewable energy sources. This framework would address cost, safety, and environmental sustainability concerns.
This framework would prioritize investment in green hydrogen production from renewable sources, offering higher incentives for projects demonstrating significant carbon emission reductions and integrating renewable energy technologies. Strict safety regulations would be implemented throughout the hydrogen value chain, from production to storage and transportation. Environmental impact assessments would be mandatory for all large-scale hydrogen projects, ensuring environmental sustainability. Furthermore, the framework would include provisions for public-private partnerships to share the risks and rewards of hydrogen infrastructure development. Finally, the government could establish a long-term strategic plan for hydrogen deployment, outlining clear targets for hydrogen production and consumption, and creating a stable policy environment to attract investment.
Closure
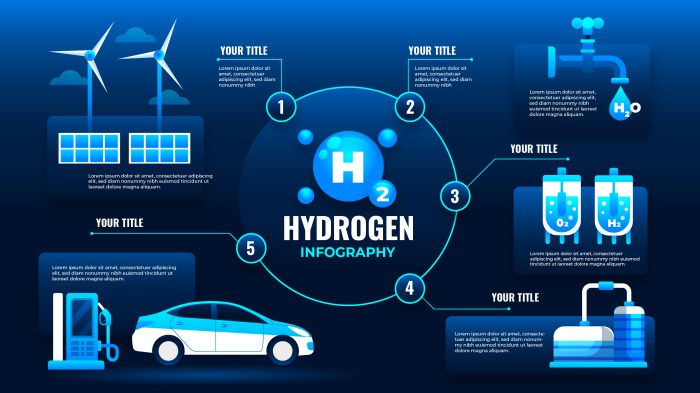
Source: readmagazine.com
In conclusion, the future of hydrogen fuel as a green energy source is brimming with potential. While significant challenges remain in terms of cost, infrastructure development, and technological advancements, the potential benefits—reduced greenhouse gas emissions, enhanced energy security, and the creation of new economic opportunities—are compelling. The concerted efforts of researchers, policymakers, and industry stakeholders are crucial to overcoming these obstacles and realizing the transformative potential of hydrogen as a clean and sustainable energy carrier.
Continued investment in research and development, coupled with supportive government policies, will be instrumental in accelerating the transition to a hydrogen-powered future.
FAQ Summary
What are the main environmental concerns associated with hydrogen production?
The environmental impact depends heavily on the production method. “Grey” hydrogen, produced from fossil fuels, contributes significantly to greenhouse gas emissions. “Green” hydrogen, produced via electrolysis using renewable energy, is environmentally friendly but requires substantial renewable energy infrastructure.
How safe is hydrogen storage and transportation?
Hydrogen is highly flammable, requiring robust safety protocols for storage and transportation. However, advancements in materials science and engineering are continuously improving the safety of hydrogen handling and minimizing risks.
What is the current cost-competitiveness of hydrogen fuel cells compared to other energy sources?
Currently, hydrogen fuel cells are generally more expensive than other energy sources like gasoline or electricity from the grid. However, costs are decreasing as technology improves and economies of scale are achieved. Government subsidies and incentives are also playing a role in reducing costs.
What are the potential long-term societal impacts of widespread hydrogen adoption?
Widespread adoption could lead to significant job creation in the hydrogen industry, improved air quality in urban areas, and reduced reliance on fossil fuels, ultimately contributing to a more sustainable and resilient energy system. However, careful planning and workforce retraining will be needed to manage the transition effectively.