Vertical Wind Turbines A Comprehensive Overview
Vertical wind turbines represent a fascinating alternative to their horizontally-aligned counterparts. Unlike traditional horizontal-axis wind turbines (HAWTs), VAWTs capture wind energy regardless of its direction, offering distinct advantages in diverse applications. This exploration delves into the design, functionality, and potential of these innovative energy harvesters, examining their various types, aerodynamic principles, and promising future applications.
From their historical development to cutting-edge advancements in materials and manufacturing, we will examine the strengths and weaknesses of VAWTs compared to HAWTs. This analysis will encompass the environmental impact, market trends, and the ongoing research shaping the future of vertical wind energy.
Introduction to Vertical Axis Wind Turbines (VAWTs)
Vertical axis wind turbines (VAWTs) represent a distinct approach to harnessing wind energy, differing significantly from their more common horizontal-axis counterparts. Their design and operational characteristics offer both advantages and disadvantages, shaping their suitability for specific applications.VAWTs are characterized by a rotor that spins around a vertical axis, as opposed to the horizontal axis rotation of horizontal axis wind turbines (HAWTs).
This fundamental difference leads to several key distinctions. The most immediately apparent is the orientation of the turbine blades; in VAWTs, they are typically positioned radially around the central axis, while in HAWTs, they are aligned along a horizontal plane. This simple difference has profound consequences for the way the turbines interact with the wind and for their overall performance characteristics.
Furthermore, VAWTs generally operate at lower rotational speeds than HAWTs, and this contributes to the overall operational characteristics and design considerations.
VAWT Design Principles and Differences from HAWTs
VAWTs employ various blade designs, including straight blades, curved blades, and more complex airfoil profiles. The aerodynamic forces acting on these blades are considerably more complex than those experienced by HAWT blades. VAWTs experience fluctuating torque and power output as the blades rotate through different wind angles, a characteristic less pronounced in HAWTs. This means that the control systems and energy capture strategies need to be tailored to the specific design and operational conditions of the VAWT.
Unlike HAWTs which primarily rely on yaw mechanisms to adjust to changing wind directions, VAWTs are inherently omnidirectional, capable of capturing wind from any direction without needing to actively orient themselves. This simplifies the mechanical design, but requires careful consideration of the aerodynamic interactions across the full range of wind angles.
Advantages and Disadvantages of VAWTs
VAWTs possess several advantages over HAWTs. Their omnidirectional nature eliminates the need for complex yaw mechanisms, resulting in simpler designs and potentially lower manufacturing costs. They are also generally better suited for urban environments and locations with complex wind patterns due to their ability to capture wind from any direction. However, VAWTs typically exhibit lower efficiency than HAWTs, particularly at higher wind speeds.
The fluctuating torque and aerodynamic complexity can also pose challenges in terms of control systems and structural design. Further, their lower rotational speeds generally mean they require larger rotors to generate the same amount of power as a HAWT.
Historical Overview of VAWT Development
The concept of VAWTs dates back to the late 19th century, with early designs focusing on simpler, Darrieus-type rotors. Significant advancements occurred in the mid-20th century, with research efforts driven by the need for alternative energy sources. The development of advanced computational fluid dynamics (CFD) modeling has significantly aided in the optimization of VAWT blade designs and performance.
While HAWTs have dominated the wind energy market, ongoing research and development continue to explore the potential of VAWTs for niche applications and specific environments, particularly in areas where their inherent advantages outweigh their limitations. Notable milestones include the development of improved blade designs, the incorporation of advanced control systems, and the exploration of hybrid VAWT-HAWT systems.
Types of Vertical Axis Wind Turbines
Vertical axis wind turbines (VAWTs) come in a variety of designs, each with its own strengths and weaknesses regarding efficiency, torque production, and overall power output. The choice of VAWT design depends heavily on the specific application and site conditions. Understanding these differences is crucial for selecting the most appropriate turbine for a given project.
Darrieus Wind Turbines
The Darrieus turbine, also known as a straight-bladed vertical axis wind turbine, is characterized by its curved blades arranged symmetrically around a vertical axis. These turbines utilize the aerodynamic lift generated by the rotating blades to produce power. Their design allows them to start up in a wide range of wind speeds, a significant advantage over horizontal axis wind turbines (HAWTs).
However, they require a starting mechanism, as they don’t self-start as reliably as Savonius turbines. Their performance is generally considered superior to Savonius turbines in terms of power output and efficiency, especially at higher wind speeds. The efficiency of a Darrieus turbine is heavily influenced by the blade design and the tip speed ratio (the ratio of blade tip speed to wind speed).
Savonius Wind Turbines
Savonius rotors are arguably the simplest type of VAWT, featuring two or more semi-circular blades arranged in a cup-like shape. Unlike Darrieus turbines that rely on lift, Savonius rotors primarily generate torque through drag. This makes them inherently self-starting and relatively robust. However, their efficiency is generally lower than Darrieus turbines, especially at higher wind speeds. Their simpler design and robust construction make them suitable for low-wind applications where reliability and ease of maintenance are prioritized over high power output.
Savonius turbines are often employed in applications requiring low-power generation, such as water pumping or small-scale electricity generation.
Helical Wind Turbines
Helical VAWTs combine aspects of both Darrieus and Savonius designs. They feature helical blades that combine lift and drag forces to generate torque. This hybrid design aims to leverage the advantages of both parent designs, offering improved self-starting capabilities compared to Darrieus turbines while potentially achieving higher efficiency than Savonius rotors. However, the design complexity can lead to higher manufacturing costs and potentially more challenging maintenance requirements.
The performance characteristics of helical turbines are still an area of ongoing research and development, with various designs and blade configurations being explored to optimize efficiency and power output.
Comparison of VAWT Types
The following table summarizes the key features of three prominent VAWT designs:
Type | Advantages | Disadvantages | Applications |
---|---|---|---|
Darrieus | High efficiency at higher wind speeds, relatively high power output | Requires starting mechanism, complex design, can be sensitive to wind shear | Larger scale power generation, hybrid systems |
Savonius | Self-starting, simple design, robust construction, low cost | Low efficiency, low power output, high rotational torque fluctuations | Small-scale power generation, water pumping, ventilation |
Helical | Combines advantages of Darrieus and Savonius, potentially higher efficiency than Savonius | Complex design, potentially higher manufacturing costs, less mature technology | Emerging applications, potentially suitable for mid-scale power generation |
Aerodynamics of Vertical Axis Wind Turbines
Understanding the aerodynamics of Vertical Axis Wind Turbines (VAWTs) is crucial for optimizing their performance and efficiency. Unlike horizontal-axis turbines, VAWTs experience constantly changing angles of attack as they rotate, leading to a more complex aerodynamic behavior. This complexity necessitates a detailed analysis of the forces at play and the influence of blade design.
Vertical wind turbines offer a promising avenue for harnessing wind energy, particularly in urban environments. However, their intermittent nature necessitates efficient energy storage to ensure a consistent power supply. This is where advancements in Renewable energy storage solutions become crucial, allowing for the reliable integration of vertical wind turbine energy into the grid. Further research into battery technologies and other storage methods will greatly enhance the practicality of these innovative turbines.
The aerodynamic forces acting on a VAWT blade are primarily lift and drag. Lift is the force perpendicular to the direction of airflow, while drag opposes the direction of airflow. The magnitude and direction of these forces vary continuously as the blade rotates, influenced by the instantaneous angle of attack – the angle between the blade’s chord line and the relative wind.
This dynamic interaction makes VAWT aerodynamics significantly different from that of horizontal-axis turbines, which operate at relatively constant angles of attack.
Blade Design Parameters and VAWT Performance
Blade design parameters significantly influence VAWT performance, impacting both the magnitude of the generated forces and the overall efficiency of energy conversion. These parameters interact in complex ways, and optimizing them often requires advanced computational techniques.
Blade shape plays a critical role. Symmetrical airfoils, while simpler to manufacture, generally exhibit lower lift-to-drag ratios compared to asymmetrical airfoils. Asymmetrical airfoils, designed with a cambered surface, can generate higher lift forces for a given angle of attack, improving overall turbine efficiency. The chord length, the distance between the leading and trailing edges of the blade, also affects performance.
Longer chord lengths generally lead to increased lift and drag forces, but can also increase the weight and manufacturing costs of the blades. Finally, the pitch angle, the angle between the blade’s chord line and the plane of rotation, influences the angle of attack experienced by the blade throughout its rotation cycle. Careful selection of the pitch angle is essential for optimizing the lift and drag forces over a complete rotation.
Computational Fluid Dynamics (CFD) Modeling of VAWT Aerodynamics
Computational Fluid Dynamics (CFD) is a powerful tool used to model and simulate the complex flow patterns around VAWT blades. CFD simulations solve the Navier-Stokes equations, which govern fluid motion, to predict the pressure and velocity fields around the rotating blades. These simulations provide detailed information about the aerodynamic forces acting on each blade element at each point in the rotation cycle.
The accuracy of CFD simulations depends on several factors, including the mesh resolution (the fineness of the computational grid used to represent the flow field), the turbulence model used (which accounts for the chaotic nature of turbulent flow), and the accuracy of the airfoil data used to define the blade shape. Advanced CFD simulations can incorporate complex phenomena such as blade-vortex interaction and wake effects, leading to more accurate predictions of VAWT performance.
Vertical wind turbines offer a promising solution for urban environments due to their smaller footprint. Optimizing their energy output, however, requires sophisticated management strategies, which is where advancements in AI in green energy management become invaluable. AI can predict wind patterns and adjust turbine operations for maximum efficiency, ultimately boosting the overall effectiveness of these innovative vertical wind turbines.
For example, a detailed CFD simulation of a Darrieus-type VAWT might reveal optimal blade shapes and pitch angles that maximize energy capture under specific wind conditions. These simulations are invaluable for designing and optimizing VAWTs for specific applications and environmental conditions, ultimately improving their efficiency and reducing their manufacturing costs.
VAWT Applications and Market Trends
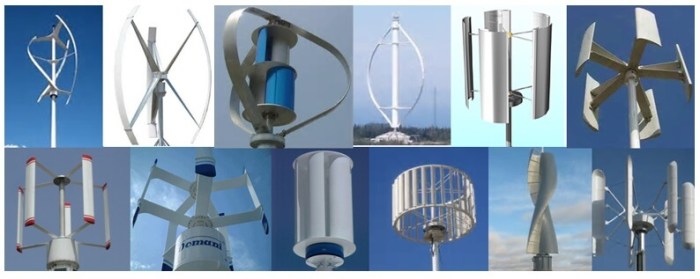
Source: windpowerengineering.com
Vertical axis wind turbines (VAWTs) are gaining traction as a viable alternative to traditional horizontal axis wind turbines (HAWTs), offering unique advantages in various applications. Their ability to operate effectively in diverse wind conditions and their relatively simple design are driving market growth and innovation. This section explores the diverse applications of VAWTs and examines the current market landscape and future projections.VAWT applications span a wide range of sectors, driven by their inherent design characteristics.
Their suitability for locations with inconsistent or low-velocity winds makes them attractive for specific uses.
VAWT Applications in Diverse Sectors
The versatility of VAWTs makes them suitable for a variety of applications, including off-grid power generation in remote areas where grid connection is impractical or expensive. Their ability to capture wind from multiple directions also makes them ideal for urban environments with complex wind patterns, where HAWTs may be less efficient. Furthermore, VAWTs are increasingly being used for water pumping, particularly in developing countries where reliable power sources are scarce.
Other applications include small-scale power generation for residential use, integration into building designs for renewable energy harvesting, and use in hybrid systems combined with solar power. Examples include remote telecommunication towers powered by VAWTs, urban street lighting powered by small-scale VAWT installations, and rural water pumping systems reliant on VAWTs.
Vertical wind turbines offer a promising avenue for sustainable energy generation, particularly in urban environments where space is limited. Their design makes them a compelling option for supplementing home energy needs, and they align perfectly with the growing interest in Green energy for homes. Further research into their efficiency and scalability could significantly enhance their role in a decentralized, renewable energy future.
Ultimately, the development of vertical wind turbines could lead to more sustainable home energy solutions.
Current Market Size and Growth Potential
The global VAWT market is currently relatively small compared to the HAWT market, but it is experiencing significant growth. While precise figures vary depending on the source and methodology, several market research reports indicate a compound annual growth rate (CAGR) of around 15-20% over the next decade. This growth is fueled by increasing demand for renewable energy, technological advancements in VAWT design and manufacturing, and government incentives promoting the adoption of renewable energy technologies.
For instance, the increasing focus on decentralized energy generation, particularly in areas with limited grid infrastructure, is boosting the demand for VAWTs. Furthermore, advancements in materials science and control systems are improving the efficiency and cost-effectiveness of VAWTs, making them a more competitive option.
Future Developments and Technological Advancements
Future developments in VAWT technology are likely to focus on improving efficiency, reducing costs, and expanding applications. Advancements in aerodynamics and blade design are expected to increase energy capture, while the use of lighter and stronger materials will reduce manufacturing costs and improve durability. Integration with smart grid technologies and energy storage systems will enhance the reliability and stability of VAWT-based power systems.
For example, the development of advanced control algorithms and the use of artificial intelligence (AI) to optimize VAWT performance in variable wind conditions is a key area of research. Similarly, the exploration of new materials like carbon fiber composites promises to significantly enhance the efficiency and lifespan of VAWT blades. The incorporation of VAWTs into hybrid energy systems, combining them with solar or other renewable sources, is another significant trend that will likely shape the future of VAWT technology.
One could imagine a future where VAWTs are seamlessly integrated into the built environment, providing clean energy to homes and businesses in a silent and efficient manner.
Manufacturing and Materials for VAWTs
The manufacturing process and material selection for Vertical Axis Wind Turbines (VAWTs) are crucial factors influencing their performance, cost-effectiveness, and lifespan. The choice of materials depends on factors such as the desired turbine size, operating environment, and required durability. Manufacturing techniques must be precise to ensure optimal aerodynamic performance and structural integrity.The selection of materials for VAWT construction involves a careful balance between strength, weight, cost, and corrosion resistance.
Several common materials are employed, each with its own advantages and disadvantages.
Common Materials Used in VAWT Construction
Steel remains a popular choice due to its high strength-to-weight ratio and relatively low cost. However, its susceptibility to corrosion necessitates protective coatings, adding to the manufacturing complexity and cost. Aluminum offers a lighter alternative with excellent corrosion resistance, although its lower strength compared to steel might necessitate thicker components, potentially impacting the overall cost and efficiency. Finally, composite materials, such as fiberglass-reinforced polymers (FRP), are increasingly used due to their high strength-to-weight ratio, design flexibility, and corrosion resistance.
These materials allow for intricate blade designs, potentially optimizing aerodynamic performance. The choice between these materials often involves trade-offs based on the specific application and design requirements.
Manufacturing Processes Involved in VAWT Blade Fabrication and Assembly
VAWT blade fabrication typically involves several stages. For steel blades, this often begins with cutting and shaping steel sheets or profiles using techniques like laser cutting, waterjet cutting, or machining. Welding or bolting is then used to assemble the components. Aluminum blades often follow a similar process, but may also incorporate casting or extrusion techniques for complex shapes.
Composite blade fabrication is more involved. It typically begins with the creation of molds, followed by the layering of composite materials (fibers and resin) according to a specific design. This is followed by curing processes, often involving heat and pressure, to solidify the composite structure. Regardless of the material, the blades are often carefully balanced to minimize vibrations during operation.
Final assembly involves attaching the blades to the central rotor shaft and integrating the turbine with its supporting structure and power generation system. Precision is paramount at each stage to guarantee the turbine’s smooth operation and longevity.
Hypothetical Manufacturing Process for a Darrieus-Type VAWT
Let’s consider a hypothetical manufacturing process for a small-scale Darrieus-type VAWT intended for residential applications. This VAWT will utilize aluminum blades for its lightweight and corrosion-resistant properties.The process would begin with Computer-Aided Design (CAD) modeling of the blades. This model would define the precise shape and dimensions of each blade, ensuring optimal aerodynamic performance. Next, CNC machining would be used to create the aluminum blade sections from extruded aluminum profiles.
These sections would be carefully inspected for dimensional accuracy. Subsequently, the individual sections would be joined together using high-strength rivets or bolts, ensuring a strong and rigid structure. The completed blades would then undergo a balancing process to minimize vibrations. The central rotor shaft, likely made from steel for its strength, would be manufactured separately using appropriate machining techniques.
Finally, the balanced blades would be attached to the rotor shaft, and the entire assembly would be integrated with a suitable gearbox, generator, and mounting structure. Quality control checks would be performed at each stage to guarantee the turbine’s structural integrity and operational efficiency. This process, while hypothetical, represents a realistic approach to manufacturing a small-scale Darrieus VAWT using readily available technologies.
The specific techniques and materials may vary depending on the scale and design of the VAWT.
Environmental Impact and Considerations
Vertical axis wind turbines (VAWTs) present a compelling alternative to horizontal axis wind turbines (HAWTs), but their environmental impact requires careful consideration. While often touted for their potential environmental benefits, a balanced assessment necessitates examining both advantages and disadvantages compared to HAWTs. This section will analyze the environmental footprint of VAWTs, focusing on noise pollution, bird mortality, and strategies for mitigation.VAWTs, due to their design and operational characteristics, exhibit a distinct environmental profile compared to HAWTs.
Their slower rotational speeds and lower blade tip speeds generally result in reduced noise pollution. However, the specific noise levels are dependent on factors such as turbine size, design, and operational conditions. Similarly, while the vertical orientation might reduce bird collisions, the potential for interaction remains, especially with smaller bird species. The impact on avian populations is a complex issue, dependent on factors like turbine location, bird migration patterns, and the effectiveness of mitigation strategies.
Noise Pollution Comparison
The lower rotational speeds of VAWTs generally lead to lower noise levels compared to HAWTs. HAWTs, with their high-speed rotating blades, produce significant broadband noise, impacting nearby communities. Studies have shown that VAWTs produce a less intense and more concentrated noise signature, potentially reducing noise annoyance. The specific noise levels, however, are highly dependent on the turbine design, operating conditions (wind speed), and the surrounding environment.
For instance, a larger VAWT operating in a high-wind environment might produce more noise than a smaller HAWT in a low-wind environment. Effective noise mitigation strategies, such as optimized blade designs and strategic siting, are crucial to minimize the impact.
Bird Mortality and Mitigation Strategies, Vertical wind turbines
Bird mortality is a significant environmental concern associated with wind energy development. While HAWTs have been shown to pose a higher risk due to their faster blade speeds and larger swept area, VAWTs are not immune to bird collisions. The lower blade speeds of VAWTs might reduce the severity of collisions, but the potential for collisions still exists, particularly for smaller birds.
Mitigation strategies are essential to reduce the risk to avian populations. These include careful site selection to avoid areas of high bird activity, employing bird deterrent systems such as flashing lights or acoustic devices, and designing turbines with features that minimize bird interactions, such as rounded blade tips. Regular monitoring and data collection are crucial for evaluating the effectiveness of these strategies.
Environmental Benefits and Drawbacks of VAWTs
A comprehensive assessment of the environmental impact necessitates considering both potential benefits and drawbacks.
- Potential Benefits: Reduced noise pollution compared to HAWTs; potentially lower bird mortality rates due to slower rotational speeds; ability to be integrated into urban environments more easily; potential for reduced visual impact in certain contexts.
- Potential Drawbacks: Potential for bird collisions, although generally lower than HAWTs; potential for noise pollution, though typically less intense than HAWTs; the manufacturing process may involve the use of materials with environmental impacts; the long-term environmental impact of materials used in VAWT construction needs further study.
Future Research and Development
The future of vertical axis wind turbines (VAWTs) hinges on overcoming current limitations and capitalizing on emerging opportunities. Significant advancements are needed to enhance their efficiency, reduce manufacturing costs, and expand their applications beyond niche markets. This requires a multi-pronged approach focusing on materials science, aerodynamic design, and control systems.Research and development efforts should prioritize improving VAWT efficiency and reducing costs.
This involves exploring novel blade designs, optimizing control strategies, and utilizing advanced manufacturing techniques. The development of more robust and cost-effective materials is crucial for widespread adoption.
Improved Aerodynamic Designs
Current VAWT designs often suffer from relatively low power coefficients compared to horizontal axis wind turbines (HAWTs). Research is focusing on innovative blade profiles and configurations to mitigate this. Computational fluid dynamics (CFD) simulations are playing a crucial role in optimizing blade geometry for maximum energy capture across a wider range of wind speeds. For instance, studies exploring the use of bio-inspired designs, mimicking the movement of natural structures like fish fins or bird wings, show promise in improving aerodynamic performance.
These designs aim to reduce drag and enhance lift, ultimately increasing the power output of the VAWT. Further research into the use of advanced materials, such as carbon fiber composites, could further enhance the aerodynamic efficiency by enabling the creation of lighter and stronger blades with optimized flexibility.
Advanced Control Systems
Optimizing the rotational speed and orientation of the VAWT in response to fluctuating wind conditions is vital for maximizing energy extraction. Advanced control systems, incorporating real-time wind speed and direction sensors, can significantly improve the performance of VAWTs. The implementation of artificial intelligence (AI) and machine learning (ML) algorithms offers a pathway to developing sophisticated control strategies that adapt dynamically to changing environmental conditions.
For example, AI-powered control systems could predict wind fluctuations and adjust the VAWT’s operation accordingly, leading to a more consistent and efficient energy output. Such systems could also account for variations in wind shear and turbulence, optimizing the turbine’s performance even in complex wind environments.
Cost Reduction Strategies
High manufacturing costs are a major barrier to the widespread adoption of VAWTs. Research is underway to explore innovative manufacturing techniques that reduce production time and material costs. The use of additive manufacturing (3D printing) techniques offers the potential to create complex blade geometries with greater precision and at lower cost compared to traditional methods. Furthermore, the exploration of less expensive materials, while maintaining structural integrity and durability, is crucial.
For example, research into the use of recycled materials or bio-based composites could lead to significant cost reductions without compromising performance. Standardization of components and modular designs can also simplify manufacturing and reduce costs. A real-world example is the development of prefabricated VAWT modules that can be easily assembled on-site, minimizing installation time and labor costs.
Expanding VAWT Applications
Beyond traditional electricity generation, VAWTs hold significant potential in various sectors. Their ability to operate effectively in low wind speeds and their relatively compact design make them suitable for integration into urban environments. For example, VAWTs could be incorporated into building facades or rooftops to generate on-site renewable energy. Furthermore, VAWTs could play a crucial role in powering remote or off-grid locations where traditional power grids are unavailable.
In the maritime sector, smaller-scale VAWTs could be used to supplement the power needs of ships or offshore platforms. Their potential for use in water pumping systems for irrigation or water purification in developing countries is also being explored. The development of hybrid systems, combining VAWTs with other renewable energy sources such as solar power, could enhance energy security and resilience in various applications.
Illustrative Examples of VAWT Designs: Vertical Wind Turbines
Vertical Axis Wind Turbines (VAWTs) come in a variety of designs, each with its own strengths and weaknesses regarding efficiency, cost, and suitability for specific applications. The following examples highlight the diversity in VAWT technology.
Darrieus Wind Turbine
The Darrieus turbine, also known as the eggbeater turbine, is one of the most common VAWT designs. Its defining characteristic is its curved, airfoil-shaped blades that rotate about a vertical axis.
Vertical wind turbines offer a promising solution for harnessing wind energy, particularly in areas with limited space. Their design makes them suitable for various locations, and their integration into rural electrification projects is gaining traction. This aligns perfectly with the broader goals of providing sustainable Green energy for rural areas , ensuring reliable power access for communities often overlooked by traditional grid infrastructure.
Ultimately, the compact nature of vertical wind turbines makes them a key player in achieving decentralized green energy solutions.
- Blade Profile: The blades typically have a symmetrical airfoil profile, allowing for efficient energy capture across a wide range of wind speeds and directions. The curvature is crucial for generating lift as the blades move through the wind. This shape maximizes the lift force generated on the blades.
- Overall Structure: The turbine consists of two or three curved blades attached to a central rotating shaft. The entire structure is typically mounted on a tower, with the shaft supported by bearings at the top and bottom. The simplicity of the design contributes to its lower manufacturing cost compared to horizontal-axis turbines.
- Mechanism of Energy Conversion: As the wind flows past the curved blades, it generates lift, causing the blades to rotate. This rotational motion is then transferred to a generator via the central shaft, converting the kinetic energy of the wind into electricity. The rotational speed is generally regulated to optimize energy output and prevent damage.
Savonius Wind Turbine
Unlike the Darrieus turbine, the Savonius rotor utilizes drag rather than lift as its primary mechanism for energy generation. This makes it simpler to manufacture but generally less efficient.
- Blade Profile: The Savonius rotor typically consists of two or three semi-circular blades, often resembling a scoop. The blades are typically not airfoil shaped, and their design is simpler and less aerodynamically sophisticated than the Darrieus design. This simplicity leads to easier manufacturing and lower cost.
- Overall Structure: The structure is relatively simple, comprising a vertical shaft with the semi-circular blades attached. The design is typically less complex and requires less precise manufacturing tolerances than the Darrieus turbine.
- Mechanism of Energy Conversion: The wind creates a pressure difference between the concave and convex sides of the blades. This pressure difference causes a torque on the shaft, driving the rotation. The rotation of the shaft is connected to a generator to produce electricity. The efficiency is generally lower compared to lift-based designs like the Darrieus due to the reliance on drag.
Helical-Axis Wind Turbine
The helical-axis VAWT represents a more recent and innovative design, attempting to combine the advantages of both lift and drag mechanisms.
- Blade Profile: The blades in a helical-axis turbine are typically helical or spiral-shaped. This unique shape allows for continuous engagement with the wind, resulting in smoother operation and potentially higher efficiency. The profile is designed to optimize both lift and drag forces, depending on the blade’s orientation relative to the wind.
- Overall Structure: The turbine features a central shaft with the helical blades extending outwards. The design often incorporates features to reduce vibrations and improve stability during operation. This can involve sophisticated structural support mechanisms and blade designs.
- Mechanism of Energy Conversion: The helical design ensures that a portion of the blade is always presenting an optimal angle to the wind, maximizing both lift and drag forces. This leads to more consistent torque and potentially higher energy capture compared to other VAWT designs. The rotational energy is then converted into electricity through a generator connected to the central shaft. The complex design requires more sophisticated modeling and analysis to optimize performance.
Last Point
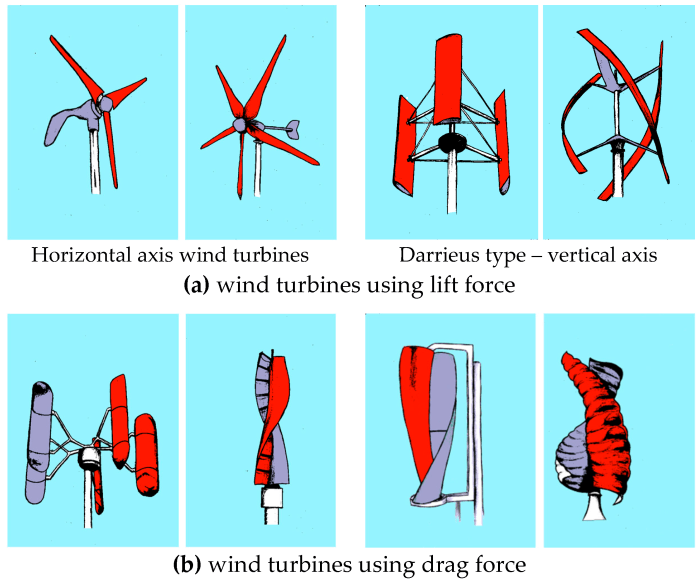
Source: mdpi.com
Vertical wind turbines, with their unique design and operational characteristics, offer a compelling solution for harnessing wind energy in various contexts. While challenges remain in optimizing efficiency and cost-effectiveness, ongoing research and development promise to unlock their full potential. The versatility of VAWTs, coupled with their adaptability to diverse environments, positions them as a significant player in the renewable energy landscape, offering a promising path towards a more sustainable future.
FAQ Section
What is the lifespan of a vertical axis wind turbine?
The lifespan varies depending on the design, materials, and maintenance, but generally ranges from 15 to 25 years.
Are VAWTs noisy?
Noise levels are generally lower than HAWTs, but specific noise output depends on design and operational parameters.
How do VAWTs perform in low-wind conditions?
While generally less efficient than HAWTs in high winds, some VAWT designs exhibit better performance in low-wind conditions.
What are the maintenance requirements for VAWTs?
Maintenance needs are comparable to HAWTs, primarily focusing on blade inspection, lubrication, and gear system checks.
Can VAWTs be used for off-grid power generation?
Yes, VAWTs are particularly suitable for off-grid applications due to their omnidirectional wind capture capability.